Analysis of Corneo-scleral Measurements and Ocular Biometry as Predictors of Scleral Lens Parameters: A Review
Purpose: In the latest years, the interest in scleral lenses is progressively increasing among practitioners and patients from all around the world. An optimal lens fit is necessary to ensure patient comfort and visual quality. However, it is not straightforward to estimate the appropriate scleral lens parameters for a particular patient. This review paper aims to summarize the current state of knowledge in predictors of scleral lens parameters based on corneo-scleral shape.
Material and Methods: Literature was reviewed from PubMed. A total of 33 articles were specifically selected for the current study.
Results: Even though not all available corneo-scleral measurements may be helpful in the fitting experience, the refractive state of the cornea, corneal flattest and steepest keratometry, scleral toricity, and axial length have proven to influence the scleral lens parameters and, consequently, the quality of scleral lens fit.
Conclusion: The usefulness of corneal, scleral, and corneo-scleral measures is reviewed and critically evaluated. A special effort was made to highlight the clinical implications of the findings.
Introduction
Initially, practitioners did not understand the advantages of scleral lenses, which represented a niche area available in a few specialized practices. Their benefits on visual quality, wide range of indications, and comprehensive education and sources allowed their spread globally. The interest in scleral lenses is increasing progressively among practitioners and patients from all around the world. Understanding the ocular surface profile is crucial for scleral lens fitting since the back surface of the lens should adequately align on the conjunctival tissue to provide comfort and guarantee ocular surface health. The evolution of technology in mapping the ocular surface has led to a better understanding of the ocular surface contour and optimizing scleral lens fitting and designs. Optical coherence tomography (AS-OCT) and corneo-scleral profilometry provide various data helpful when fitting scleral lenses. However, their use is restricted to a few clinical practices in only limited countries, while corneal topography has become increasingly available in contact lens practice worldwide.
Since the scleral lens vaults the cornea and rests exclusively on the conjunctiva, it may be expected that the assessment of corneal topography is not necessary when fitting scleral lenses as it does not provide accurate information for scleral lens parameters. Regarding the corneal zone, the main challenge is estimating the appropriate vault to avoid a corneal touch or an excessive vault that can compromise corneal physiology. Knowing the corneal sagittal height at a particular chord is thus essential. Although the earlier recommendation consisted in choosing the base curve of the first diagnostic scleral lens 1.00 D steeper than the steepest corneal curve of 48.00 D if reliable keratometry or corneal topography were not available, or beginning with the base curve that closely matches the reference sphere from a topographic map, it has been shown that not all corneal parameters can predict scleral lens parameters.1
Contrary to the cornea, the sclera significantly impacts lens design since the scleral lens rests exclusively on the conjunctival tissue that overlies the sclera. A non-optimal fitting relationship affects lens centration, visual outcomes, and patient comfort.
Moreover, some studies related to myopia focused on the sclera as it is a tissue that expands significantly during myopia progression.2,3 The sclera undergoes continuous remodeling throughout life,3,4 and its biomechanical characteristics are crucial in determining the size and shape of the eye, consequently, the refractive state of the eye.3 Studies have demonstrated that the scleral shape significantly correlates with the refractive error 5-8 and axial length.5,9,10 Thus, it may be thought that the amount of myopia and ocular biometry may potentially predict scleral lens parameters.
This article reviews the current state-of-the-art research works that aim to establish whether corneal measurements can predict scleral lens parameters, determine how scleral profile influences scleral lens design, and focus on scleral shape correlation with refractive errors and axial length and their implication in scleral lens parameters. The corneal diameter and limbal width are not considered in this work, but they will influence the ideal scleral lens specification prescribed.
Method of research
The literature was reviewed from PubMed using the following keywords, separately and in combination: Human scleral shape (510 results), scleral profile (493 results), scleral shape correlation (70 results), scleral topography (302 results), scleral contour (105 results), scleral asymmetry (79 results), corneal asymmetry (621 results), corneal topography and scleral lens parameters (27 results), scleral shape in keratoconus (19 results), scleral shape and myopia (107 results), scleral shape and axial length (61 results). From all the articles found, a total of 33 articles were explicitly selected for the current study. The selection criteria were articles focused on the studies of the corneo-scleral shape in the human eye and its relationship with scleral lens parameters.
Technology to map the corneo-scleral contour
Different technologies have been used to measure the corneo-scleral profile.11 However, only a few of them, such as Anterior-Segment Optical Coherence Tomography (AS-OCT) and corneo-scleral topographers (Eye Surface Profiler (ESP), sMap3D, and Pentacam), reached clinical interest. The AS-OCT is a high-resolution cross-sectional imaging modality specially designed for anterior segment imaging.12
The ESP and sMap3D are height profilometers able to measure the corneo-scleral topography up to ≈ 18.00 – 20.00 mm horizontally and slightly smaller chord vertically. The ESP uses a best-fit sphere model to provide the measurements of the eye heights achieving similar levels of accuracy for corneal surface heights as Placido disc-based videokeratoscopes, with below 10 μm root mean squared error (RMSE) for the central 8.00 mm area of a calibrated artificial surface, and below 40 μm for an extended measurement area of 16.00 mm.13 sMap3D measures the ocular surface with, according to the manufacturer, 10 μm precision. The measurements in sMap3D are taken in primary, superior, and inferior gaze directions. The software then stitches the three images and creates a single topography. It is worth noting that the reported resolution information, taken from manufacturers of the different measuring devices should not be directly compared, as one is given in terms of RMSE while the other remains elusive.
In addition, and even though Scheimpflug cameras have been traditionally restricted to corneal analysis, recently, Oculus Pentacam carries an optional software, the Cornea Sclera Profile (CSP) modulus, to assess the corneo-scleral profiles up to 18.00 mm. Pentacam Scheimpflug technology consists of a blue light slit illumination and a decentered camera with a Scheimpflug objective to tilt the focal plane to the same level as the focus of the slit illumination. The optical media (corneal layers, crystalline lens) scatter the light, and corresponding backscattered light can be captured using a Scheimpflug camera. CSP is also equipped with a link to external scleral lens fitting software.
Albeit non-Scheimpflug corneo-scleral topographers have proven to provide consistent and repeatable measurements in corneal and scleral sagittal height 14,15 and offer consistent measurements at the corneal level with Pentacam HR readings,16 recent research showed that scleral sagittal height and toricity measurements measured with ESP and CSP Pentacam could not be considered as interchangeable.17
Corneal measurements and scleral lens parameters
Corneal topography and scleral lens base curve
Schornack et al. first investigated the relationship between corneal topographic indices and scleral lens base curve.1 All patients were fitted with standard 18.20 mm Jupiter scleral lenses, which were among the first scleral lenses widely available and were defined by the base curve. The earlier definition of scleral lenses by the base curve allowed for a softer transition from corneal to scleral lenses. While Schornack et al. found a positive correlation between the steep and flat K power, they concluded that topographic indices could not accurately predict the base curve of an adequately fitted scleral lens.1 Corneal topography may potentially be helpful to determine the corneal sagittal height of the scleral lens at a certain chord.
Corneal sagittal height and scleral sagittal height in the abnormal cornea
Macedo-de-Araújo et al. compared the corneal shape with the parameters of the scleral lenses of various diameters (ranging between 15.20 and 16.40 mm) that were fitted in 126 eyes presenting different corneal conditions: primary corneal ectasia, penetrating keratoplasty, post-surgical ectasia, and regular corneas with high refractive errors (myopia ≥ 6.00 D; hyperopia ≥ 4.00 D and astigmatism ≥ 2.00 D).18 They observed a weak correlation between the scleral lens sagittal height and the corneal profile assessed with a corneal topographer at 10.00 (ρ ranging from 0.38 to 0.45) and 12.00 mm (ρ ranging from 0.39 to 0.46). In contrast, they found a moderate correlation (ρ ~ 0.60 ) when comparing the scleral lens sagittal height with the ocular estimated height (EH-Chord) calculated for the diameter of the lens that subjects were wearing. The EH-Chord parameter was derived from the corneal topographer (Medmont Studio 6.1), and it provides the sagittal height of the anterior eye surface for a chord value set by the clinician. The mean differences between the EH-Chord and scleral lens sagittal height were 447 ± 290 μm, 391 ± 260 μm, and 389 ± 360 μm for EH-Chord 0 – 180º, 150 – 330°, and 30 – 210°, respectively. The large standard deviation indicates high variability. For clarity, 447 ± 290 means that for 2/3 of the population the difference between the two parameters varies from 157 to 780 µm and for 95 % of the population from 0 to 1070. Consequently, the association has poor relevance clinically. These differences were attributed to the scleral lens vault at the cornea. However, the difference varied between meridians, and the correlation between these two parameters was higher at 30 − 210°. Therefore, the authors recommend calculating a vault of 389 μm when a 16.40 mm scleral lens is fitted, predicting the best trial lens by measuring the EH-Chord for 16.40 mm. These outcomes are in agreement with those of a pilot study that assessed the use of the AS-OCT for designing and fitting scleral lenses finding a strong correlation between the corneal sagittal height and that of the scleral lens.19
Other reports have suggested the use of corneal sagittal height provided by the corneal topographer to estimate the initial diagnostic scleral lens sagittal height.20,21 To the corneal sagittal height calculated by the topographer at chord 10.00 mm (Figure 1), it is necessary to add 200 μm height for every additional 0.50 mm chord. For example, if the corneal sagittal height at 10.00 mm is 1910 µm, as in Figure 1, and the sagittal height of the scleral lenses in the diagnostic is calculated at 16.50 mm. To reach a chord of 16.50 mm from the original 10.00 mm chord we need to add 2600 µm. These is calculated considering an increase of 200 μm for every additional 0.50 mm chord (i.e., 16.50 − 10.00 = 6.50; 6.50 ÷ 0.50 = 13 additional 0.5 mm-chord; 13 additional 0.5 mm-chord × 200 μm ÷ 0.5 mm-chord = 2600 μm). The sum results in a total of 4510 µm height at 16.50 mm (1910 µm + 2600 µm = 4510 µm). Then it is necessary to consider the vault over the corneal surface. Considering lens settlement, the initial vault may be considered 350 µm. Thus, referring to this method, the estimated scleral lens sagittal height for a cornea with a sagittal height of 1910 μm at 10.00 mm is 4860 µm (4510 µm + 350 µm = 4860 µm).

Corneal keratometry and landing zone radius
Corneal keratometry (K) readings may predict the trend of the scleral landing zone. A significant correlation was found between corneal curvature and scleral radii (R2 = 0.42, p < 0.001).22 A larger corneal radius corresponds to a greater scleral radius in healthy eyes.7,22 This correlation was stronger in emmetropic eyes.22 Thus, a flatter landing zone is required to avoid lens compression on the conjunctival tissue in these cases.
In eyes with keratoconus, the sclera tends to steepen as the cornea steepens16,23,24 R2 = 0.84, p < 0.001.16 A steep landing zone will avoid lens edge lifting off and lens decentration.
Corneal profile and scleral lens peripheral design
Corneal steepening can help predict the scleral profile and the location of the steepest scleral angle.16 With corneal steeping, the cornea becomes more irregular, influencing the scleral shape, which will get more irregular16 (Figure 2). Eyes with different conditions (primary corneal ectasia, penetrating keratoplasty, post-surgical ectasia, and regular corneas with high refractive errors) were assessed with corneal topography. They were divided into two main groups: Group 1 included eyes with irregular cornea and Group 2 eyes with regular cornea presenting high refractive errors.18 The authors found no relationship between corneal astigmatism and the peripheral toricity of the scleral lenses fitted in the irregular cornea group. However, in group 2, such correlation was evident. Eyes where the scleral lens required higher peripheral toricity also had greater corneal toricity (more than 36 μm at 10.00 mm, and 69 μm at 12.00 mm). For further investigations, subjects of group 2 were subdivided into sub-groups: the first subgroup included eyes with low corneal toricity, and the second had eyes with high corneal toricity (≥ 2.00 D). The results confirmed that higher corneal toricity corresponded to a greater amount of toricity needed in the scleral lens haptic. Also, the level of cone decentration in advanced cases of ectasia may predict the need for a toric landing zone16,24 (Figure 3).
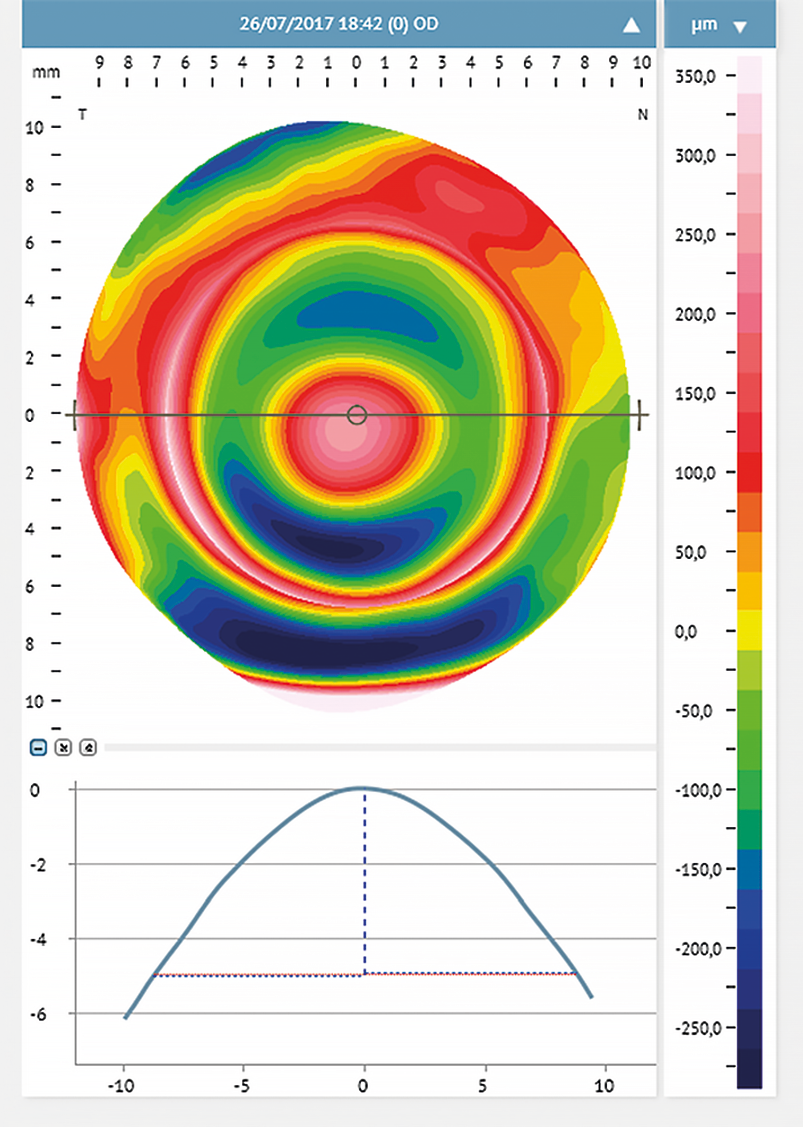
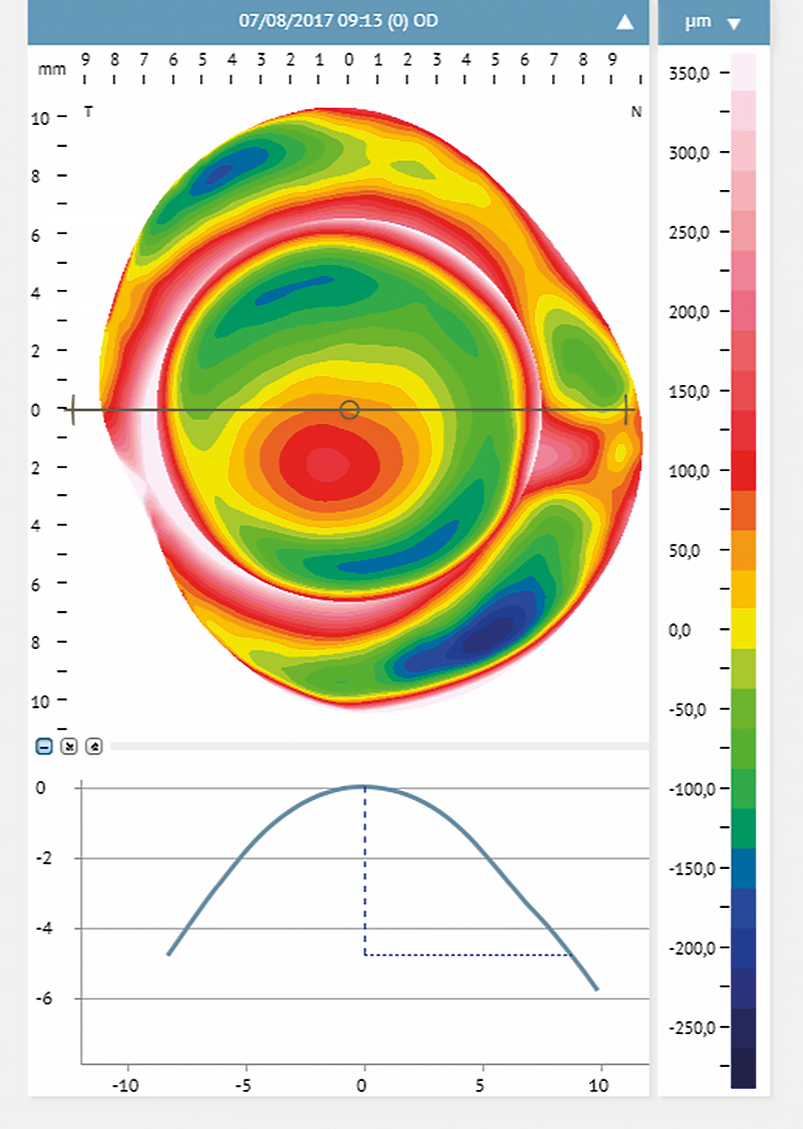
Summary:
- There is a weak correlation between the scleral lens sagittal height and the corneal profile.
- Scleral lens sagittal height is correlated with the ocular sagittal height at a given chord (Figure 4).
- Corneal steepening will indicate a more likely toric or irregular sclera.
- In eyes with irregular astigmatism, corneal astigmatism is not correlated to scleral toricity.
- In eyes with regular astigmatism, there is a strong correlation between corneal toricity and lens haptic toricity. Higher corneal toricity corresponds to a greater amount of toricity needed in the scleral lens landing zone.
Clinical implications:
- Corneal topography may help predict scleral lens peripheral parameters and design.
- Some corneal topographers provide the corneal sagittal height at chord 10.00 mm. This value can be used to estimate the initial diagnostic scleral lens sagittal height.
- Knowing the ocular sagittal height is important for the initial diagnostic scleral lens selection.
- Corneal topography can be used to predict the need for a toric landing zone in irregular corneas; however, it cannot be used to predict the characteristics of the lens.
- Fitting scleral lenses in emmetropic eyes may require a flatter landing zone if the cornea exhibits a large radius.
- High corneal toricity indicates the necessity of a scleral lens with a toric landing zone. The higher the corneal astigmatism, the greater toricity is needed in the lens periphery.
- Fitting scleral lenses in eyes with keratoconus, the lens may need a steeper landing zone to avoid lens decentration.
- In advanced ectasia, with decentered cone, scleral lenses may benefit from a toric, quadrant-specific, or customized landing zone.
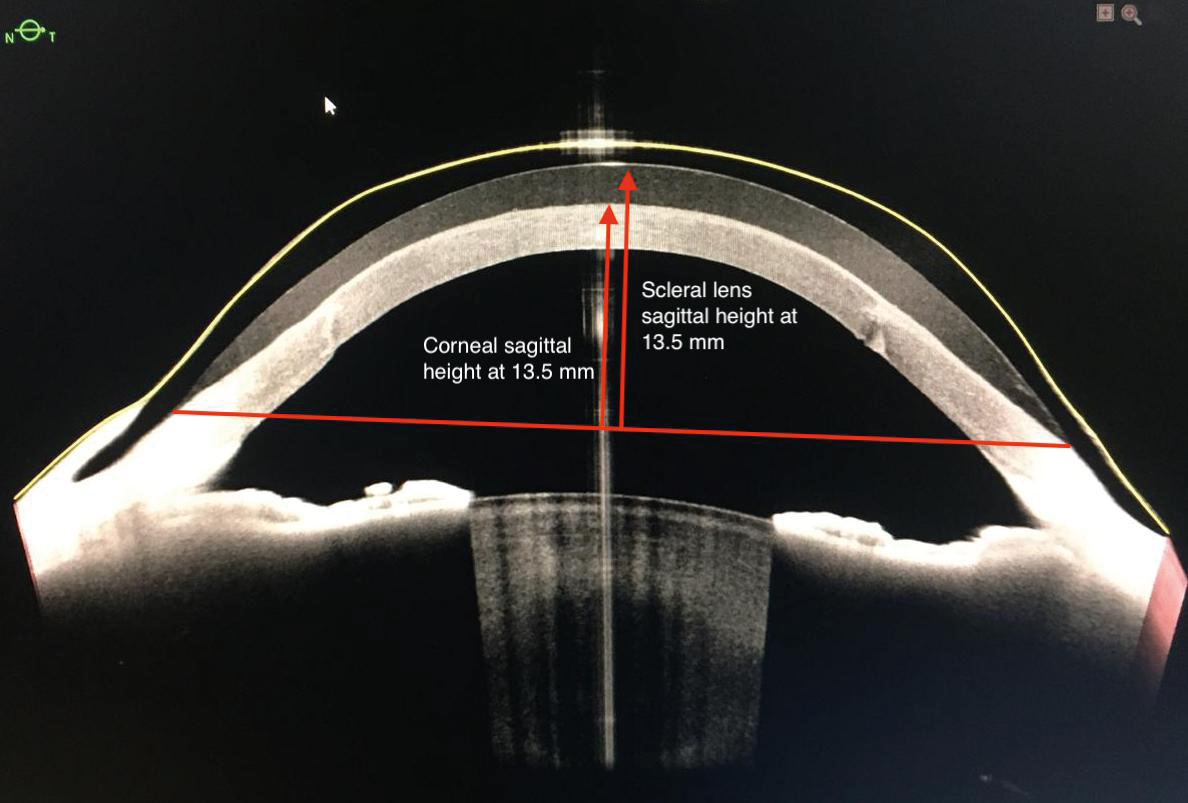
Scleral topography and scleral lens parameters
Scleral asymmetry and scleral lens toricity
Gemoules first used the AS-OCT cross-sectional image to assess the peripheral corneal and scleral curvature to design customized scleral lenses.25 Similarly, Ritzmann et al. used the AS-OCT to determine the anterior scleral shape and its role in scleral lens design.26 They showed that scleral asymmetry at chord 15.00 mm or beyond is significant, suggesting adding peripheral toricity to large scleral lenses to achieve an optimal alignment with the underlying tissue and provide more comfort. Le et al. also evaluated the correlation between scleral toricity and the scleral lens landing zone toricity using the AS-OCT.19 Although they found a modest correlation and the sample size was small (including six eyes), the authors concluded that the AS-OCT data might help determine scleral lens fitting.
Scleral asymmetry and lens decentration and compression
As the scleral nasal hemisphere is significantly flatter than the temporal, a spherical scleral lens will first touch the most elevated nasal area and tend to move toward the opposite less elevated quadrant to find equilibrium. This will result in temporal scleral lens decentration with a non-uniform distribution of the corneal peripheral clearance: the nasal segment will present less clearance, while in the temporal segment, there will be excessive clearance.26 Scleral lenses with quadrant-specific designs or customized periphery are indicated in eyes exhibiting scleral asymmetry. These results are in agreement with Consejo et al.,27 who assessed the relationship between scleral asymmetry and scleral lens compression on the conjunctival tissue using an ESP. The authors found that scleral asymmetry may predict scleral lens settling and decentration. Scleral asymmetry was significantly positively correlated with lens decentration and (r = 0.71, p = 0.002) compression (r = 0.81, p < 0.001). Furthermore, decentration and compression were positively correlated, indicating that larger decentration will induce greater compression. Thus, the larger the scleral asymmetry is, the more the lens will be displaced, causing increasing compression. Lens decentration was larger in the horizontal meridian than in the vertical, and it was greater temporally.
Changes in conjunctival elevations and scleral lens design
Conjunctival irregularities
Conjunctival elevations such as pinguecula, pterygium, glaucoma filtration devices, or drainage implants negatively affect the scleral lens fitting success as they may cause impingement and conjunctival staining in the specific area where they are evident (Figure 5). Lens edge interaction with the irregularity may cause conjunctival inflammation.28,29 A notch, localized peripheral areas of increased elevation, quadrant-specific design, or customized haptic may be indicated
in these cases.
Progression or treatment of keratoconus
Recently, it has been reported that corneal collagen cross-linking30 and corneal hydrops31 may cause a substantial change in the scleral elevation profile and asymmetry, from 100 to 400 μm,30,31 which may affect the scleral lens fit. These scleral elevation changes may be due to structural changes in scleral tissue. A standard toric scleral lens would exhibit a sub-optimal fitting. Customized scleral lenses are indicated in these cases.
Summary:
- Scleral asymmetry increases significantly at chord 15.00 mm and beyond.
- A spherical lens touches the flattest scleral area and tends to decenter towards the opposite steepest area.
- Scleral asymmetry may predict scleral lens settling and decentration.
- Scleral asymmetry has a significant positive correlation with lens decentration and compression location.
- Lens decentration and compression are positively correlated.
- Conjunctival irregularities represent a challenge when fitting scleral lenses.
- Corneal collagen cross-linking and corneal hydrops may cause a substantial change in the scleral elevation profile and asymmetry.
Clinical implications:
- Scleral lenses with a diameter of 15.00 mm or greater necessitate a toric or asymmetric landing zone.
- The scleral lens tends to exhibit a temporal decentration inducing a non−uniform distribution of the corneal peripheral clearance.
- In high corneal asymmetry, scleral lenses with quadrant-specific designs or customized periphery are indicated.
- The higher the scleral asymmetry is, the greater the lens decentration causing increasing compression.
- A notch, localized peripheral areas of increased elevation, quadrant-specific design, or customized haptic are indicated in case of irregular conjunctiva.
- When corneal collagen cross-linking and corneal hydrops induce a high level of scleral asymmetry, customized scleral lenses are indicated.
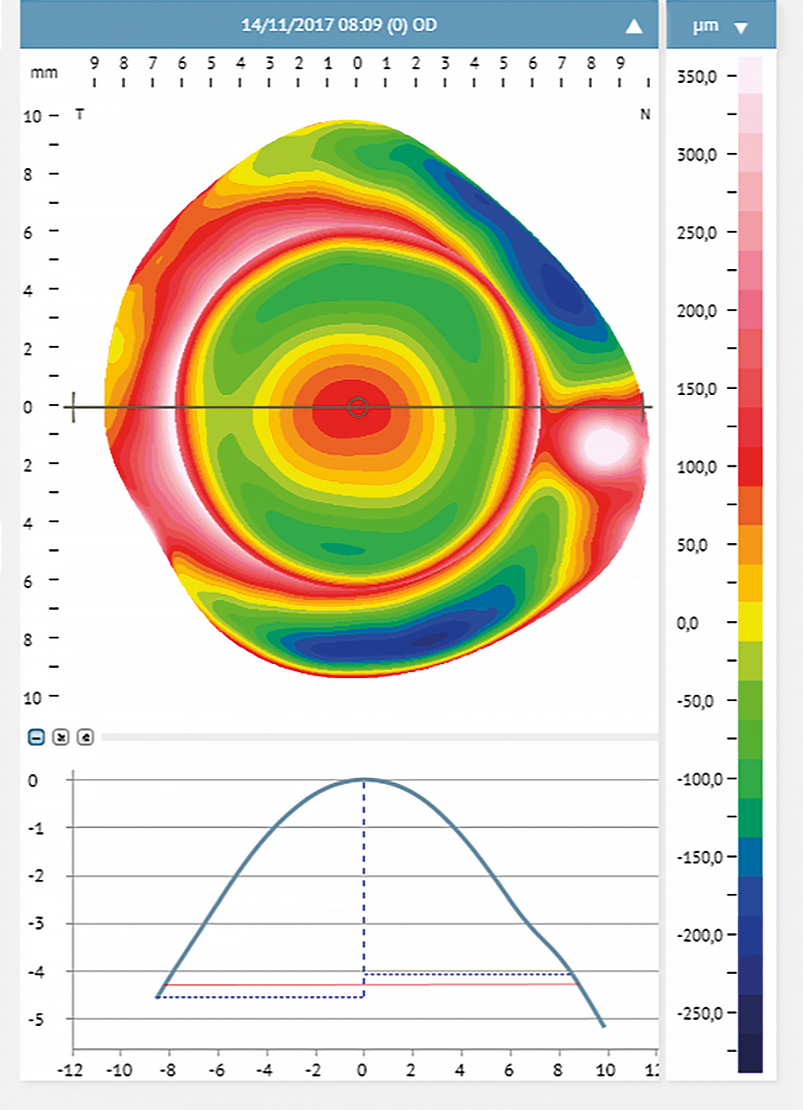
Myopic refractive error and anterior scleral shape
Myopia and anterior scleral shape
The scleral profile appears to be correlated to myopia. There is a strong correlation (r = 0.76, p < 0.001) between myopia and scleral axial radius curvature; with myopia increase, the sclera becomes flatter, especially in the temporal area.9 Consejo and Rozema compared the scleral morphometry in emmetropes and myopes.5 They classified emmetropes as subjects with refractive errors between −0.50 D and +0.75 D, with a mean of 0.43 ± 0.5 D. Myopic participants had a refractive error ranging from −0.75 D to −8.25 D with a mean of −3.60 ± 2.3 D. In this study, the scleral asymmetry was calculated using a differential elevation map between a 3.00 D scleral topography exported from the ESP and a fixed reference surface. This automatic method grades scleral asymmetry in μm; a low value indicated a more likely regular sclera while a high value corresponds with an irregular sclera. The researchers found that the ocular sagittal height at a chord between 12.00 mm and 16.00 mm was greater in emmetropes than in myopes, and scleral asymmetry was significantly different between myopes and emmetropes, even though a considerable overlap between the two distributions in scleral asymmetry was observed. Scleral asymmetry was smaller in myopes (asymmetry ranging from 17.00 μm to 43.70 μm) than in emmetropes (asymmetry ranging from 21.00 μm to 70.30 μm).
A prospective study also compared anterior scleral shape in emmetropes (from ± 0.50 to –0.25 D; mean –0.07 ± 0.12 D) and myopes, subdividing myopia into low to moderate (from –0.50 to –5.75 D; mean –2.59 ± 1.2 D) and high myopes (from –6.00 to –10.00 D; mean –7.30 ± 1.2 D).6 The researchers measured the sagittal height at 0.70 mm from the limbus in the temporal area and 0.70, 1.40, and 2.10 mm from the limbus in the nasal area. The sagittal height and axial radius of curvature measurements from the central horizontal meridian of each average ESP map were further analyzed using external software. Similar to Consejo and Rozema,5 the authors of this study found a significant negative correlation between spherical equivalent refraction and scleral sagittal height asymmetry. Emmetropes present greater asymmetry than low myopes, and the increasing myopia is related to a reduction in scleral sagittal height asymmetry.6 Also, the mean scleral sagittal height was lower in emmetropes than in high myopic subjects at all nasal areas but at none in the temporal region. Referring to the authors, this difference may be because, with myopia progression, the scleral asymmetry decreases, as there is a greater posterior movement of the anterior surface in the nasal area than the temporal area.
Contrary to the outcomes of these studies,5,6 Piñero et al. found no correlation between spherical equivalent error and sagittal height nasal-temporal asymmetry.7 This may be due to the low to moderate range of myopia (mean of myopia −1.36 ± 2.37 D) of the subjects included in the latter study, which was not sufficient to demonstrate a significant difference with emmetropes.
Regarding the clinical aspects when fitting scleral lenses, it may be stated that the amount of myopia can help infering the scleral lens peripheral design. In eyes with high myopia, the sclera is flatter, especially in the temporal area, thus scleral asymmetry is decreased. Along with these considerations, when fitting eyes with high myopia, the scleral lens periphery may be flatter and spherical.
Axial length and anterior scleral shape
Studies showed a strong correlation between axial length and the anterior scleral shape, whether using an AS-OCT9 or ESP5,10 for the scleral assessment. Lee et al., investigating the correlation between axial length and anterior scleral curvature, found a positive correlation between axial length and scleral axial radius of curvature; the greater the axial length, the flatter the sclera, especially in the temporal area.9 Consejo and Rozema found that axial length in emmetropes ranged from 21.60 to 24.90 mm, with a mean scleral asymmetry of 42.10 ± 13.3 μm in the right eyes and 41.40 ± 13.4 μm in the left eyes. In contrast, in myopes (mean refractive error −3.50 ± 2.1 D), axial length was greater and ranged from 23.60 to 26.50 mm, with a significantly lower mean scleral asymmetry of 28.50 ± 7.2 μm in the right eyes and 26.30 ± 5.7 μm in the left eyes.5 Bataille et al. found similar outcomes assessing patients who majorly were emmetropes and myopes (a mean sphere of −1.58 D).10 The sagittal height measurements were taken at different chords: 12, 13, 14, and 15 mm. The authors found a strong negative association between the axial length and the difference between temporal and nasal sagittal heights at all chords.
This negative correlation may be explained by the fact that the sclera tends to remodel itself throughout life, depending on myopia development and elongation of the globe.32 It has been recently shown that progressive myopia caused alteration in biomechanical properties of the corneal and scleral areas.2 Since the sclera facilitates eye elongation, various strategies for myopia control were proposed to strengthen the sclera with scleral reinforcement and collagen cross-linking.33 This is crucial as a better knowledge of the anterior scleral shape in myopes may assist in determining the best myopia management strategy for every patient, considered individually.5
As myopia progresses, the eye gets more elongated and the sclera becomes flatter, particularly in the temporal area. Thus, scleral morphology becomes similar in the nasal and temporal areas and is more likely symmetrical. With this in mind, in elongated eyes, the scleral lens landing zone needs a flat radius and may be spherical.
Summary:
- With myopia progression, the sclera becomes flatter, especially in the temporal area.
- Compared to emmetropes, myopic eyes present lower scleral asymmetry. With the flattening of the temporal area, the scleral morphology gets close to the nasal quadrant. Thus, the scleral asymmetry decreases.
- The higher myopia, the lower the scleral asymmetry.
- There is an association between the axial length and scleral shape; the sclera becomes flatter as the axial length increases.
- There is a negative correlation between axial length and scleral asymmetry. The greater axial length, the lower scleral asymmetry.
- As the axial length becomes longer, the scleral temporal sagittal height decreases, and the nasal sagittal height increases.
Clinical implications:
- In high myopes, contact lenses tend to have better centration.
- When fitting scleral lenses, it is crucial to consider the spherical equivalent error. In patients with high myopia, scleral lenses may be spherical in the periphery.
- Better knowledge of the anterior scleral shape of myopic patients may aid in identifying the best myopia management strategy for each patient individually
- When fitting scleral lenses, it is crucial to consider the axial length. In patients with elongated eyes, scleral lenses may be flatter and spherical in the periphery.
Conclusion
Not all corneo-scleral measurements may be helpful to determine scleral lens parameters. The corneal profile is not correlated to the scleral lens sagittal height, while the latest parameter is positively associated with the ocular sagittal height at a particular chord. A flat cornea may indicate the necessity of a flat landing zone, while in eyes with keratoconus, corneal steepening will indicate a steeper and toric or irregular sclera. In regular corneas, high corneal astigmatism suggests the need for a great amount of toricity in lens haptic. A scleral lens of 15.00 mm or greater necessitates a toric or asymmetric landing zone. A quadrant-specific design or customized periphery are most indicated as in most eyes, the sclera is more likely asymmetric, especially in eyes with keratoconus and those undergone corneal collagen cross-linking or had corneal hydrops. Irregular conjunctiva may benefit from a notch, localized peripheral areas of increased elevation, quadrant-specific design, or customized haptic.
The ocular surface profile is also correlated to myopia and axial length. With myopia progression and increased axial length, the ocular surface gets flatter, especially in the temporal area. Thus, the elevation difference between the nasal and the temporal regions decreases; the higher myopia and the larger the axial length, the lower the scleral asymmetry. This may be explained by the fact that the sclera tends to continuously remodel its shape depending on myopia progression and globe elongation.
Further investigation is needed regarding scleral shape role and eye elongation. As for corneal cross-linking, the sclera may be strengthened to prevent the lengthening of the globe. It is also essential to explore the importance of the anterior scleral shape assessment in myopic patients to individualize the best myopia management strategy for every single patient.
Figure Copyright by Daddi Fadel.
COE Multiple Choice Questionnaire
The publication "Analysis of Corneo-scleral Measurements and Ocular Biometry as Predictors of Scleral Lens Parameters: A Review" has been approved as a COE continuing education article by the German Quality Association for Optometric Services (GOL). The deadline to answer the questions is 01.October 2024. Only one answer per question is correct. Successful completion requires answering four of the six questions.
You can take the continuing education exam while logged in.
Users who are not yet logged in can register for ocl-online free of charge here.