Current status of the topography of the sclera: a literature review
Purpose: Although a major part of the anterior surface of the eyeball is formed by the sclera, there is little confirmed data on topography of the sclera compared to the cornea. The goal of this paper was to provide an update of relevant literature published on anatomy and topography of the anterior sclera.
Methods: A systematic literature search was conducted in PubMed using the key words “scleral” and “topography.” 66 of the 310 papers dealt with the topic and were used for this present review. In addition, 5 articles from the German-language non-peer-reviewed literature were included.
Results: Several studies have demonstrated the utility of modern measurement and examination techniques, such as optical coherence tomography (OCT), Scheimpflug imaging, or Fourier-based profilometry in the assessment of scleral parameters. These allow a more comprehensive understanding of the structure and shape of the anterior sclera, simplified contact lens selection, prediction of lens fit, and assessment of changes in scleral topography. The shape of the sclera can be influenced by factors such as age, refraction, accommodation, convergence, thickness, stiffness, modulus of elasticity, intraocular pressure, keratoconus and contact lens wear.
Conclusion: A comprehensive understanding of the biometric properties and topography of the sclera of the anterior segment of the eye is an essential component for both the design and fitting of scleral as well as soft contact lenses.
Introduction
In addition to the medical history of the patient, a slit lamp examination and an analysis of the tear film, corneal topography is one of the essential measurements that are carried out before fitting contact lenses.1 The selection of the posterior surface design of rigid contact lenses is traditionally based on the central corneal radii measured with a keratometer. More advanced methods such as Placido-based topography and Scheimpflug tomography allow for a more detailed description of the shape of the central, paracentral and peripheral cornea.2 The use of corneal topography when fitting rigid lenses results in optimised lens fitting, reduced fitting times, higher success rates on the first fit, greater patient satisfaction and a reduced need for the use of trial lenses, reducing the potential risk of infection.3,4,5,6 While corneal topography is essential in selecting and predicting the fit of a rigid contact lens, there is little or no correlation between corneal topography and the fit of soft contact lenses.7,8 Soft, mini-scleral and scleral contact lenses are placed on the anterior scleral region and, thus, differences in the anterior ocular surface in this area can affect lens fitting and be relevant to the design of this type of contact lenses (Figure 1).9 A detailed insight into the topography of the anterior sclera is also important, since the total ocular sagittal height is determined not only by the central corneal radii, the corneal diameter and the corneal eccentricity, but also by the profile of the sclera.
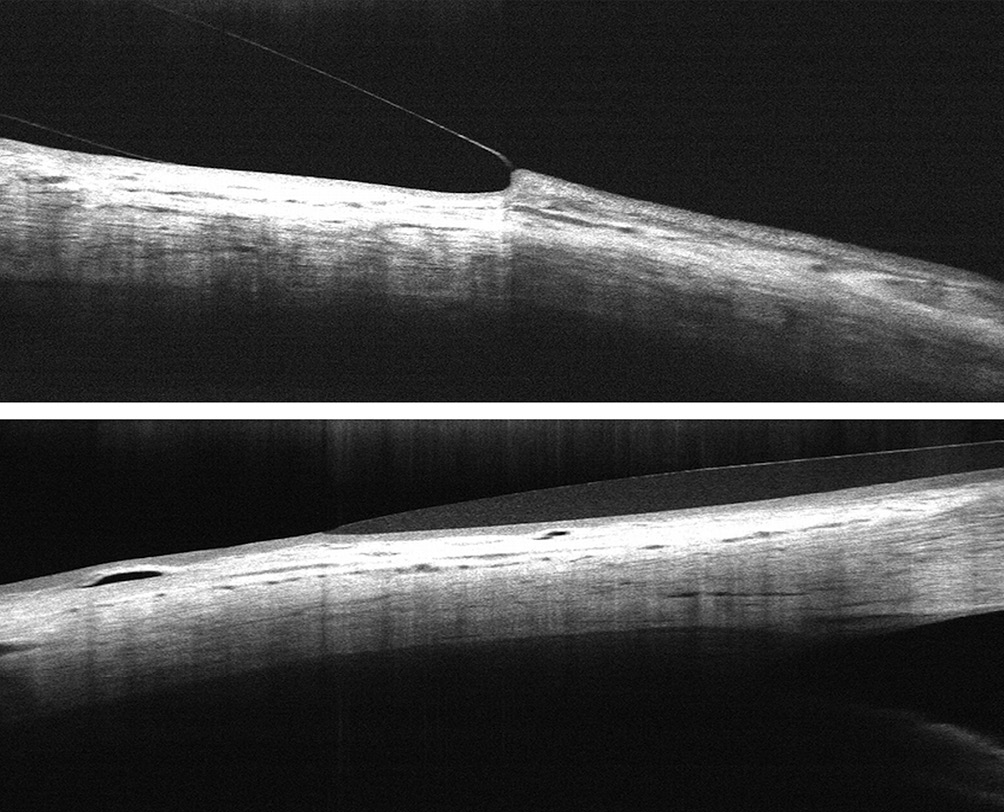
Anatomy and physiology of the sclera
The opaque sclera covers most of the surface of the bulbus oculi and ranges in thickness from 0.3 mm just behind the insertion of the recti muscles of the eye to 1.0 mm near the optic nerve.10 The anterior scleral thickness varies significantly between quadrants and resembles the spiral of Tillaux that describes the insertions of the four recti muscles.11 The sclera consists of three major layers, which are, from outermost to innermost, episclera, stroma, and lamina fusca.10 The episclera is the outermost layer of the sclera, lying between Tenon's capsule and the scleral stroma.12 It consists primarily of loosely arranged collagen bundles and is approximately 15-20 μm thick at the limbus.12 The episclera has a rich vascular supply from the anterior ciliary arteries at its junction with the fascia sheet of the eyeball, while the posterior ciliary arteries supply blood to the equator and posterior episclera.13 In contrast to the episclera, the scleral stroma is avascular, although numerous arteries supplying other structures of the eye perforate the stromal tissue.12 The stroma is the largest tissue layer of the sclera and determines its biomechanical properties. The material properties of the stroma can be summarised as nonlinear viscoelastic and result from the composition and organisation of the collagen-rich extracellular matrix.13 The stroma consists mainly of dense and, compared to the episclera, thicker collagen bundles of variable fibril diameter in a random arrangement.10 The lamina fusca, bordering the uvea, consists of shorter collagen bundles and an increased number of elastic fibers.10 The sclera on the fundus of the eye appears to have a looser arrangement of collagen fibrils, less collagen, and a lower modulus of elasticity than the anterior and equatorial sclera.14 The sclera helps determine the size and shape of the eye and consequently plays an important role in determining and maintaining its refractive status. As a robust shell, it protects the sensitive inner eye structures, such as the retina and the optic disc, from mechanical injuries. Furthermore, the tissue provides a firm attachment point for the extraocular muscles. The biomechanical properties of the sclera, and thus the tissue's resistance or susceptibility to deforming forces, such as those stemming from contact lens wear, vary with age, ethnicity, intraocular pressure, and refraction.12 Consequently, these factors can affect the fit of contact lenses placed on the sclera and viceversa: the anterior sclera can be affected by contact lenses. Exact knowledge of the topography of the sclera can therefore help to optimise contact-lens fit and avoid or minimise tissue compression in the contact area.
Scleral parameters
Various parameters have been suggested in the literature to describe the profile of the anterior sclera or the corneo-scleral junction (Figure 2). The corneo-scleral profile (CSP) describes the profile line formed by the shape of the cornea, the sulcus and the further outline of the sclera.15 The corneo-scleral junction angle (CSJ) is the angle formed between a tangent to the cornea and a tangent to the sclera in the limbal region.16 The scleral angle refers to the angle that the anterior sclera makes with a horizontal chord perpendicular to the centre of the cornea or the pupil.17 The sagittal scleral height (SAG) is the distance from a tangent at the corneal vertex to a parallel horizontal chord at the sclera.17 The scleral radius (SR) corresponds to the radius of a circle best fitted to the shape of the sclera.18
Different types of topography of the sclera
Subjective classification of the corneo-scleral profile
The influence of the corneo-scleral profile on the fitting of soft contact lenses was first described in German-speaking countries by Gaggioni and Meier in 1987.15 For ease of use on the slit lamp, the authors classified the CSP into five different profile shapes (Figure 3). This classification is intended to give the fitter information as to which contact lens design or which contact lens material is the most appropriate. In practice, the CSP can be assessed with the help of a slit lamp, a hand-held magnifying glass or even with the bare eye. However, Bokern et al.19 reported low accuracy and repeatability of this subjective classification method using the slit lamp. These results were confirmed by a recently published study by Bergmann et al.20, which showed poor intra-observer reliability (Kappa=0.098) for the subjective classification of the CSP on Scheimpflug camera images.
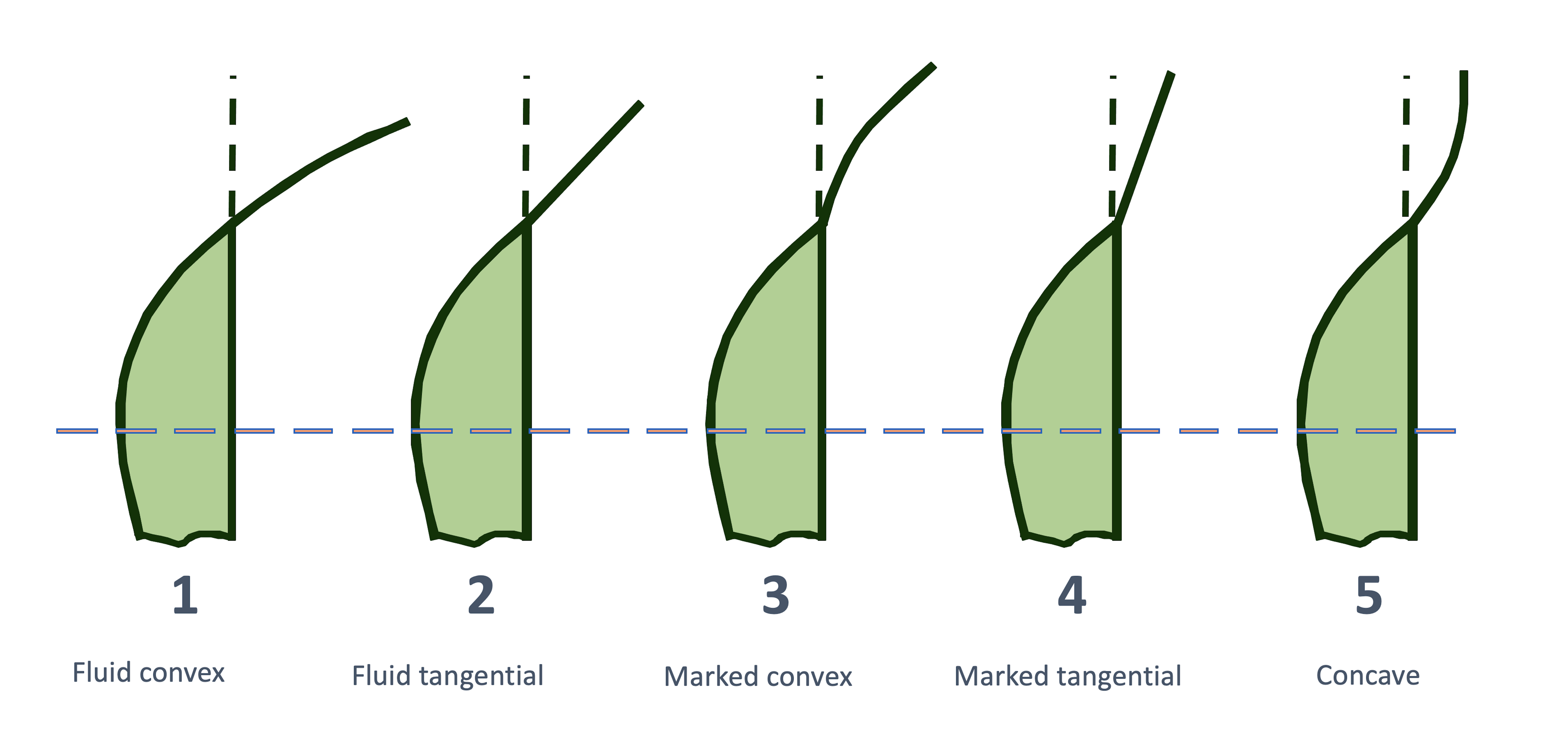
Fourier-based profilometry
Fourier transform profilometry is a contactless, 3D measurement method in which a Ronchi ruling is projected onto a diffuse three-dimensional surface and the resulting deformed image is captured by a CCD camera and processed by a computer.21 Based on this measurement principle, Jongsma et al.22 presented the prototype of a corneal topographer under the name "Maastricht Shape Topographer". This apparatus can be used to record the shape of the entire anterior surface including the sclera. Two corneo-scleral topographers with a similar measuring principle are now on the market: the Eye Surface Profiler (Eaglet Eye, The Netherlands) and the sMap3D corneo-scleral topograph (Visionary Optics, USA). These devices can measure ocular surface topography up to a diameter of 22 mm using fluorophotometry, which requires the application of fluorescein.23 With the Eye Surface Profiler, two blue line patterns are projected onto the surface of the eye from two different positions and their superimposition is then captured by a camera (Figure 4).24 A single shot is taken while the test subject is looking straight ahead. In contrast, the sMap3D uses a single blue line pattern, which is projected onto the ocular surface from a central position and then recorded by two offset cameras.25 The cameras take three pictures: one with the patient looking straight ahead, one looking up and one looking down. The three images are then combined.26 In addition to determining the parameters known from corneal topographers (central corneal radii, eccentricities, corneal astigmatism, corneal diameter, pupil diameter), Fourier-based profilometry with these measuring devices also enables the automatic measurement of the scleral sagittal heights, the scleral angles, and the scleral radii for the extended measuring range of up to 22 mm (Figure 4).
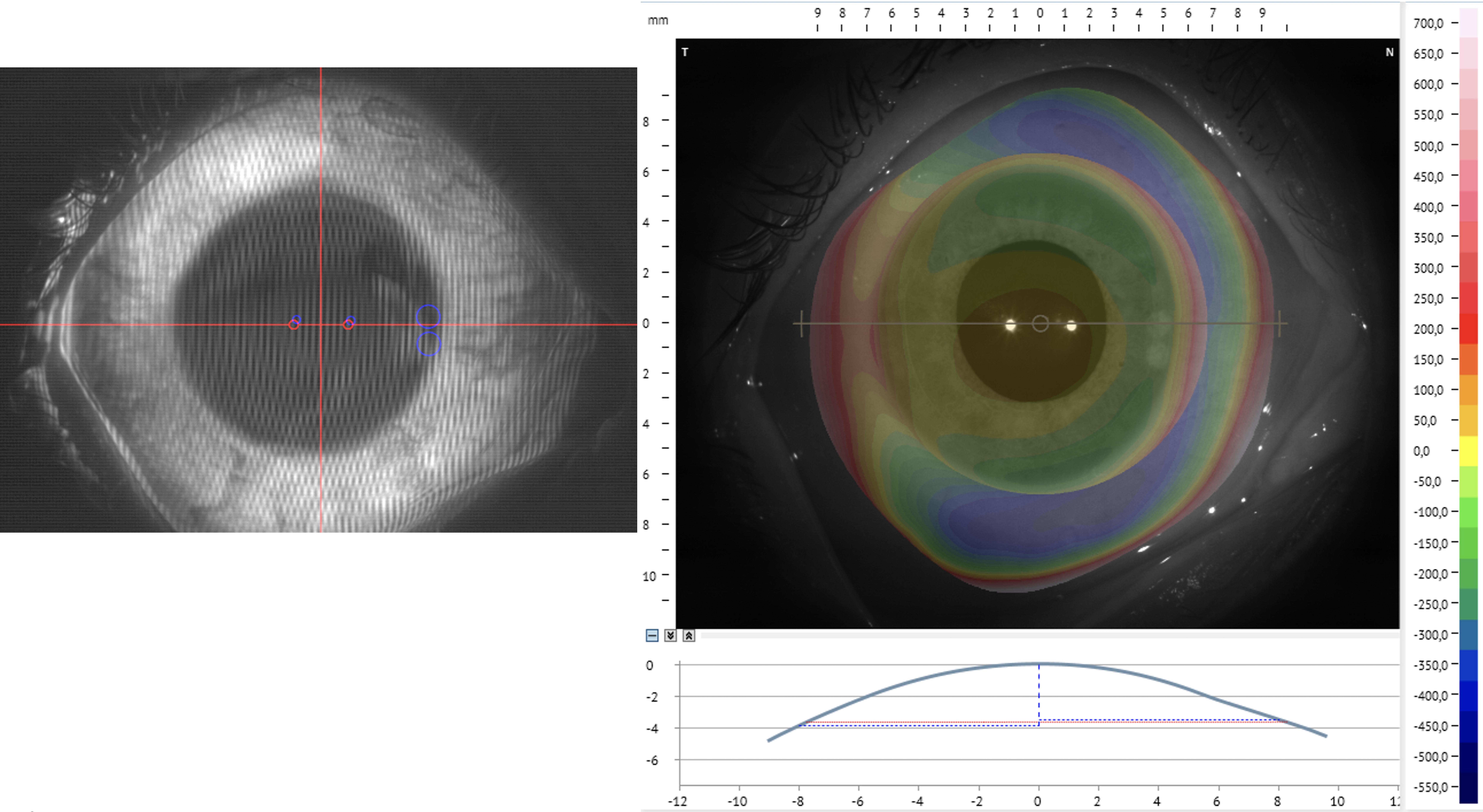
Optical Coherence Tomography (OCT)
Coherence is a property of waves that enables stationary interference phenomena. Optical coherence tomography systems analyse echo time delay measurements of backscattered or back reflected light using an interferometer with a mechanically scanned optical reference path.27 The high-resolution, in-vivo images of optical coherence tomography are increasingly being used in the anterior segment of the eye to assess the ocular surface, the tear film and the fit of contact lenses.18,28,29 Optical coherence tomography allows for a more comprehensive and detailed representation of the anterior segment as well as the peripheral corneo-scleral profile. First attempts to measure the scleral angle on OCT images were made by van der Worp et al. in 2010.30,31 In the meantime there have been numerous other studies in which, in addition to the scleral angles, the scleral radii and the scleral sagittal height were measured on OCT images of the anterior segment of the eye.16,18,32,33,34,35,36 Determining the scleral parameters using OCT, however, always requires a manual measurement using external software (Figure 5). Additionally, only individual meridians can be evaluated, so that the measurements do not result in an automatically generated topographical map of the entire anterior sclera.
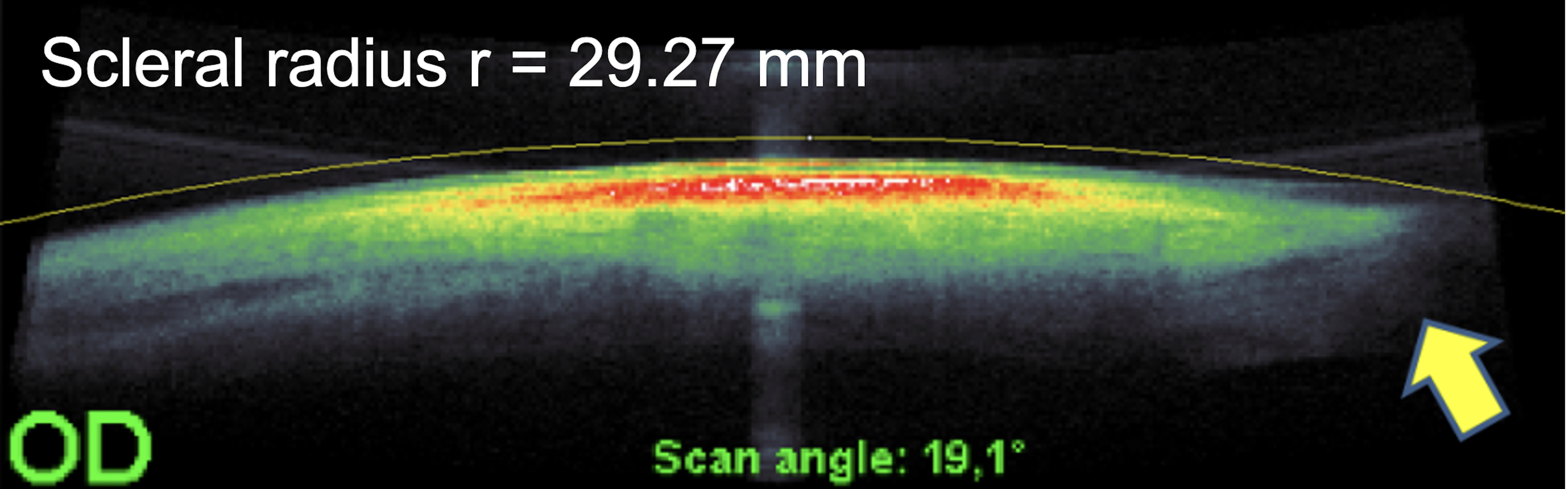
Scheimpflug cameras
The Scheimpflug technique uses three imaginary planes perpendicular to each other (lens, image and subject) instead of the two planes of a conventional or normal camera. The three planes result in an extension of the depth of field, which enables a sharp resolution of the image. Devices with a rotating Scheimpflug camera assess not only the cornea, but the entire anterior segment, ranging from the anterior corneal surface to the posterior lens surface.27 First attempts to measure the scleral radii using Scheimpflug photography were described by Tiffany et al.37 as early as 2004. A new corneo-scleral profile software module for the Pentacam (Oculus, Wetzlar, Germany) has recently been introduced to the market.24,38,39 The corneo-scleral profile module uses Scheimpflug imaging to measure sagittal height up to a chord length of 18 mm. One central and four peripheral Scheimpflug images (nasal, temporal, superior and inferior) are required to acquire a corneo-scleral profile in 25 meridians (Figure 6). Both the scleral sagittal heights and the scleral angles are measured automatically. A manual measurement of other parameters, such as the corneoscleral junction angle, is possible using external software to evaluate the Scheimpflug images.20
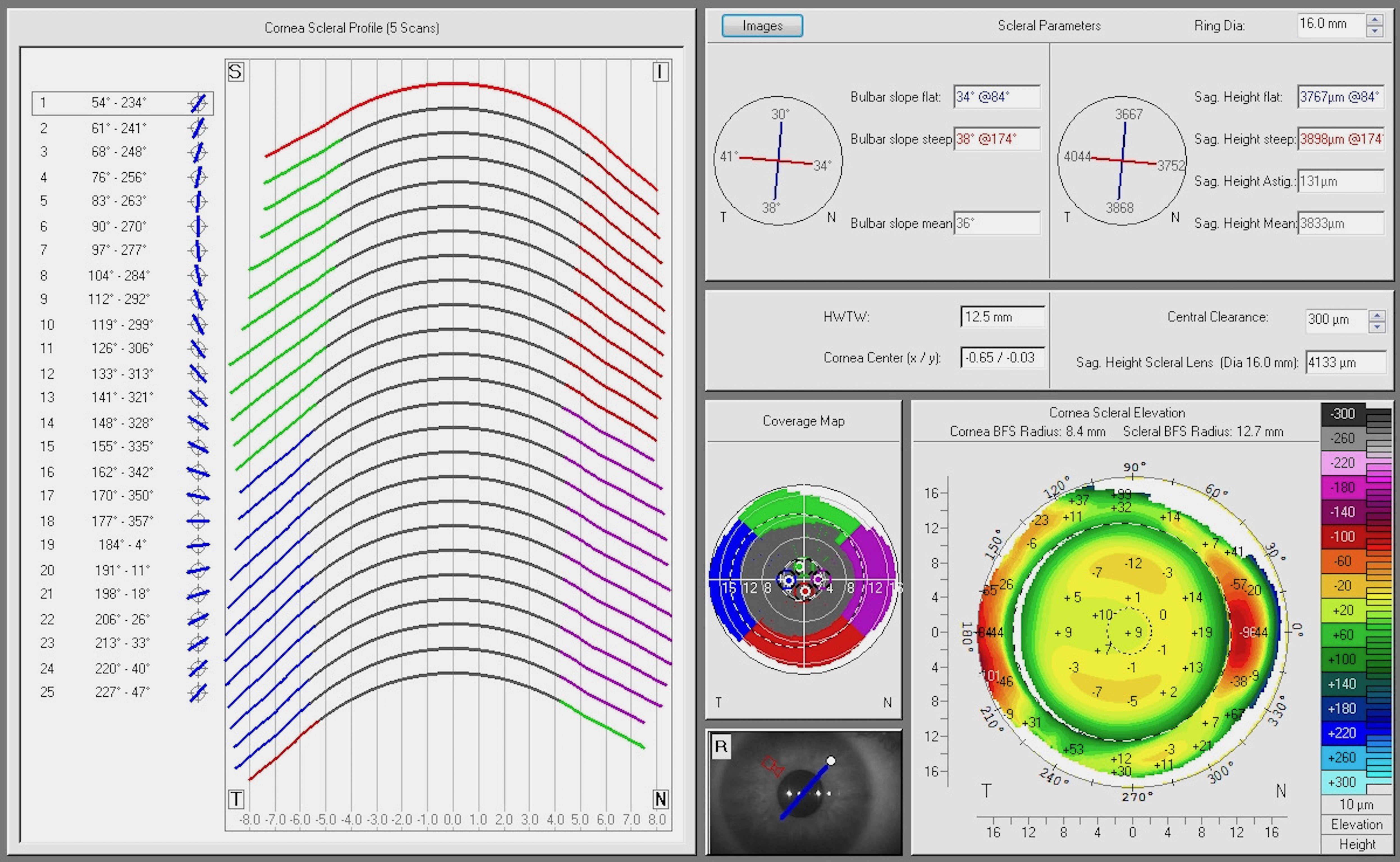
Scleral topography in healthy eyes
CSP profile
A study by Rott-Muff et al. showed in 2001 that a fluid tangential profile was the most common (42%) when classifying the CSP on the slit lamp.40 This was followed by a marked convex (28.1%), a fluid convex (22.8%) and a marked tangential (6.8%) transition. A concave-convex transition was almost non-existent at 0.3%. Comparable values were found by Bergmann et al. 2021 in the classification of the CSP on images from a Scheimpflug camera (Table 1). Here, too, the fluid tangential outline was the most common with 47.8%.20 According to this, the junction between the cornea and sclera seems to be mostly fluid without any marked transition.
Scleral angle
The scleral angle for a diameter (chord length) of 15 mm can have values between 30° and 47° (Table 2).30,31 Nasal scleral angles are smaller than temporal ones. This asymmetry of scleral angle along the horizontal meridian increases with increasing distance from the limbus.33,39
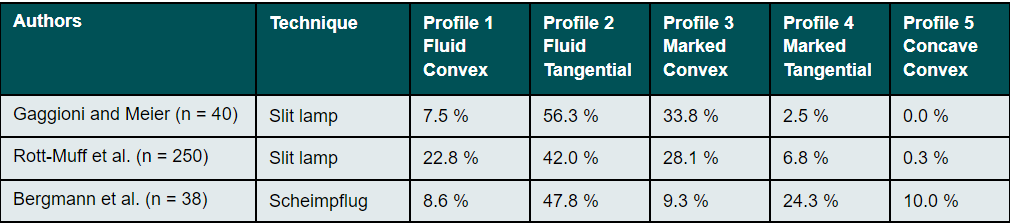
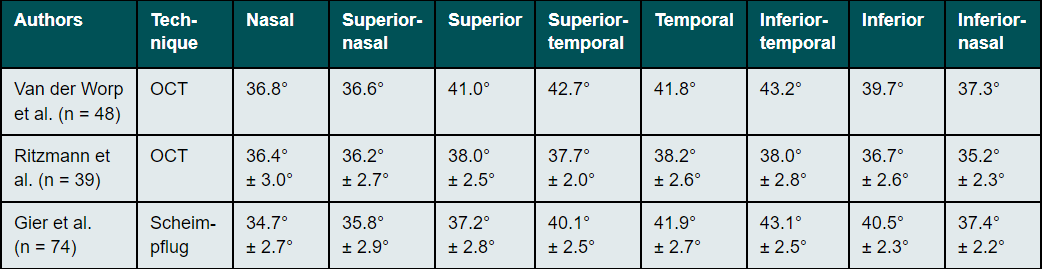
Scleral radii (SR)
The curvature of the anterior sclera can be determined invasively using impression techniques and non-invasively using the Scheimpflug technique, profilometry, and optical coherence tomography. All techniques have shown that the scleral shape appears to be more tangential and slightly curved (Table 3).18,32,33,41,42 The nasal radii of the sclera are flatter than the temporal radii16,18,32 and the asymmetry and toricity of the sclera is less pronounced near the limbus than further away from it.31,33,42,43 Differences in the anatomy of the nasal and temporal angles of the eye44, differences in the attachment points and in the width of the ocular muscles45 and variations in scleral thickness are assumed to account for the difference between nasal and temporal profiles.11,46
Corneoscleral Junction Angle (CSJ)
The radius of curvature of the anterior sclera is much flatter than that of the peripheral cornea. Thus, the outline of the sclera in the area of the limbus mostly continues almost as a straight line without any significant transition. This was already observed in early studies by Meier and Rott-Muff on the slit lamp as a fluid tangential profile and confirmed by OCT and Scheimpflug measurements.32,33,40,47 The angle formed at the junction between the cornea and the sclera comes closest to a tangent in the superior area and is most pronounced in the nasal region (Table 4).32,33,40,47 The greatest meridional asymmetry is usually observed between the temporal and nasal regions.32,33,40,47
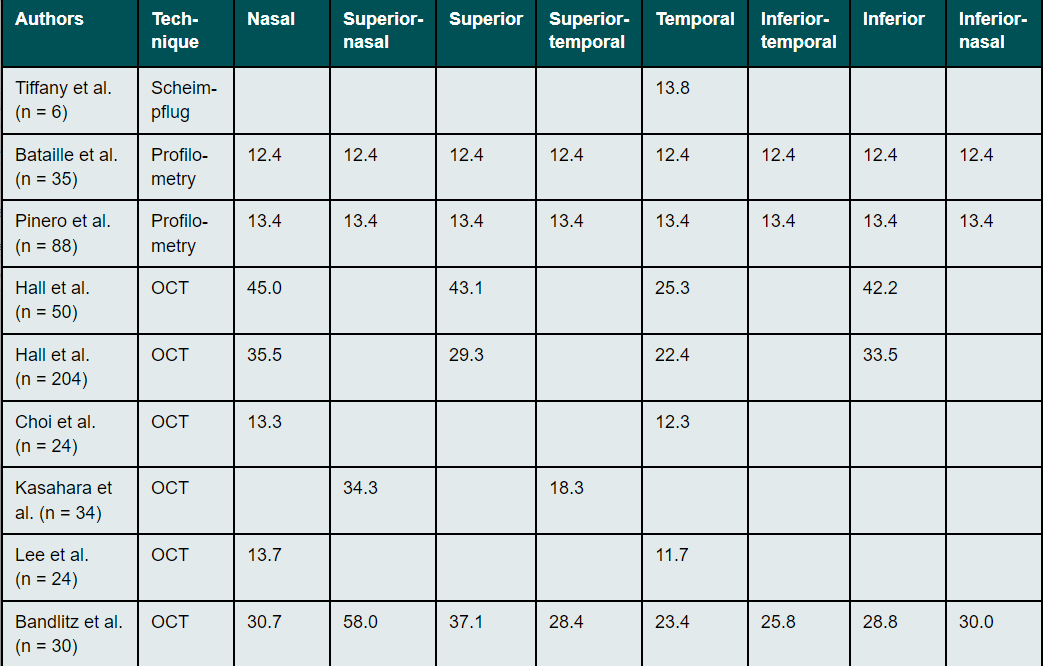

Scleral sagittal heights
Scleral sagittal heights are an important factor when selecting and manufacturing a first scleral trial lens.23 In addition, it is known that the selection of the optimal posterior surface radius of a soft contact lens does not correlate with the central corneal radii, but rather is determined by the total sagittal height of the corneo-scleral area.48,49 While corneal radii, eccentricities, and toricity can be reliably quantified using topographers, these central corneal measurements often correlate poorly with scleral sagittal heights or toricity.18,50 Consequently, the fit of scleral lenses and soft lenses cannot be predicted from a corneal measurement alone. Scleral sagittal heights can be determined using profilometry, optical coherence tomography, or Scheimpflug methods, although the measurement methods are not considered completely equivalent.24,38 For an equal chord length, the scleral sagittal heights measured by the Scheimpflug method are greater than those measured by profilometry, while the opposite is true for scleral toricity (Table 5).24,38 Consistent with the shallower scleral radii in the nasal region, scleral sagittal heights also appear to be greater in the nasal region as in the temporal one.42,51 The further away from the limbus, the greater the difference in sagittal height between nasal and temporal regions.52 Scleral toricity, which is defined as the greatest difference in sagittal height between two perpendicular meridians for a given chord length, also increases with distance from the limbus.53
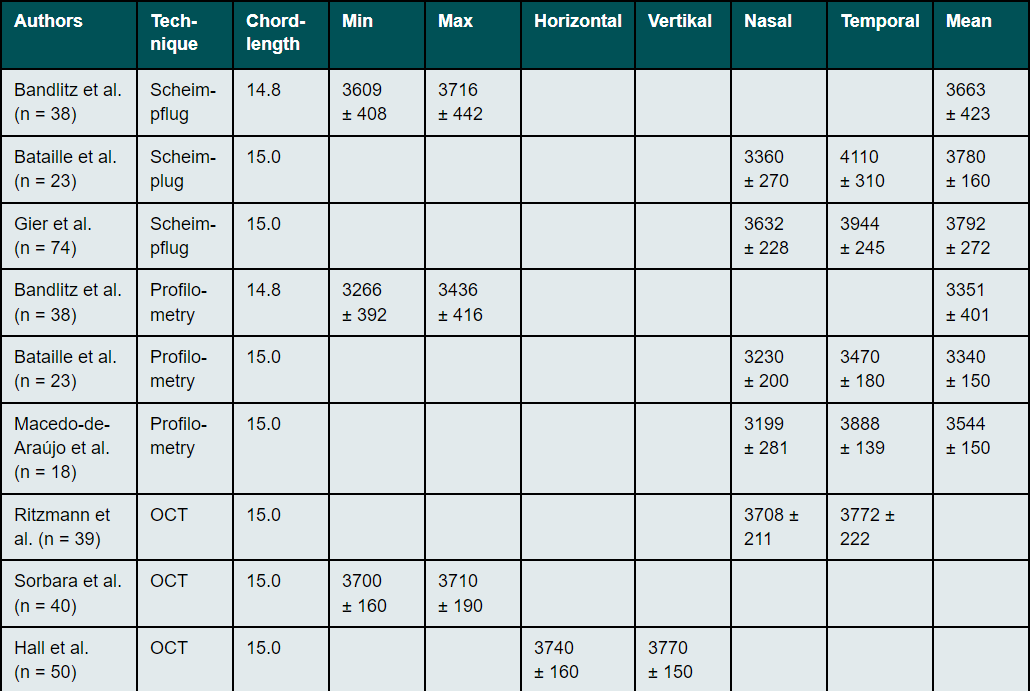
Factors affecting scleral topography
Age
Hall et al. were able to show in a study that the age of the test subjects caused a variance in the scleral topography variables.32 This was reflected in a decrease in scleral radii and corneoscleral junction angle: the scleral radii therefore appear to become smaller with age and the transition from cornea to sclera more pronounced.32 An age-related increase in scleral collagen networks, despite a reduction in overall collagen content and a decrease in glycosaminoglycan, is most likely the cause of scleral tissue stiffening with age.17 The ability of the sclera to expand is related to connective tissue hydration, which also decreases with age.17 In addition, an increase in the thickness of the sclera with age has also been reported.11,46,54,55
Intraocular pressure
The sclera protects the inside of the eye and serves to maintain intraocular pressure (IOP). The anterior sclera could therefore be a potentially interesting factor in the pathogenesis of glaucomatous optic neuropathy, since IOP-induced deformation of the sclera could be transmitted to the optic disc tissue. Given the possible impact of tissue compression on the area where scleral lenses are placed, several studies have examined the changes in intraocular pressure associated with scleral lenses wear.23 The possibility of an increase in intraocular pressure due to scleral lens wear is discussed. One hypothesis is that scleral lenses, due to their mass and size, may compress the episcleral veins and decrease aqueous humour outflow in susceptible individuals.56 Another possibility is that scleral lenses can flatten the ocular surface and displace intraocular fluid, increasing IOP.57 However, a recently published study by Walker et al.58 suggests that scleral lenses have a very minimal effect on IOP during wear and an insignificant effect on optic disc morphology in healthy adult eyes. Furthermore, Nguyen et al. were able to show that the corneal deformation response under air-jet induced deformation is significantly influenced by the properties of the sclera.59 The stiffer the sclera, the greater its influence on the deformation properties of the cornea.59
Refraction
A high degree of myopia is associated with progressive and excessive distension of the eyeball, which may be associated with degenerative changes in the sclera.55 Some studies have demonstrated geometric changes in the anterior sclera with increasing myopia. Consejo et al. showed that the asymmetry of the scleral surface decreases with increasing myopia.60 The more myopic the eye, the less asymmetric the anterior sclera is. These authors also found that the shape of the anterior sclera correlated well with the length and refractive power of the eye, and that the length of the eye could be predicted by determining parameters of the anterior sclera.60,61 Niyazmanda et al. also showed that the nasal-temporal asymmetry of scleral sagittal heights and scleral radii is less in patients exhibiting a high degree of myopia compared to emmetropes.62 Dhakal et al. found significant thinning of the anterior sclera of the inferior meridian compared to the other three meridians with increasing degree of myopia.63
Keratoconus
The topography of the sclera is of particular importance in eyes with keratoconus, since keratoconus is the most common indicator for scleral lens fitting worldwide.64 The shape of the sclera of patients with keratoconus appears to be more irregular and steeper than in healthy eyes.53,65,66 In contrast, a significantly flatter scleral shape was found in a group of Marfan syndrome patients compared to healthy eyes, which could indicate a more centrifugal stretching of the globe.67 The more advanced the keratoconus, the higher the asymmetry of the sclera appears to be.66 While the shape of the sclera in healthy eyes can only be predicted to a limited extent on the basis of the corneal topography18, the corneal parameters in patients exhibiting keratoconus seem to correlate with the asymmetry of the sclera.68 Dhaese et al. showed that when keratoconus is present, the steepest area of the central cornea, peripheral cornea, and sclera tend to share a common direction.68 In contrast, corneal astigmatism showed only a weak correlation with the degree of scleral irregularity, while posterior corneal surface curvature and corneal thickness did not correlate with scleral asymmetry.68
Accommodation and convergence
Woodman-Pieterse et al. reported significant thinning of the temporal anterior sclera during accommodation, which was more pronounced in myopes than in emmetropes.69 A change in scleral sagittal height during accommodation was also reported. This decreased in the nasal region by 390 ± 330 μm during accommodation, while it increased in the temporal region by 380 ± 280 μm.70 Niyazmanda et al. demonstrated that both accommodation and a simulated convergence affect the shape of the nasal anterior sclera, with the greatest changes being during convergence and most pronounced in the more peripheral nasal scleral regions.71 Significant forward movement and flattening of the nasal scleral surface was observed with accommodation, convergence, and their combination. These changes did not differ significantly between patients with a low- to moderate-grade myopia and emmetropes.71
Contact lenses
The anatomy of the sclera in the anterior segment of the eye can influence the fit of contact lenses placed on the sclera and vice versa. This can lead to a change in both the thickness and the topography of the sclera. Jandl et al. found the nasal part of the conjunctiva to have a larger impression when wearing soft contact lenses than the temporal part and concluded that the flatter curve of the underlying nasal sclera is responsible for this tighter fit.29 A greater nasal compression of the conjunctival and scleral tissues has also been reported after wearing scleral lenses.72 The extent of a change in the scleral sagittal height is strongly linked to the degree of asymmetry of the sclera. A higher asymmetry leads not only to greater tissue compression but also to greater decentration of a spherical scleral lens. This also means that tissue compression can be reduced if the geometry of the scleral lens is based on the geometry of the sclera in the different quadrants. Furthermore, significant flattening of the upper sclero-conjunctival surface has been demonstrated in healthy eyes after 3-5 hours of scleral lens wear, something which has been attributed to upper eye lid forces.60,73 The findings of higher scleral asymmetry in keratoconus eyes suggest that scleral lenses with a spherical placement zone are likely to result in uneven compression in different sectors.66 Rather than smoothing the scleral surface, regular wear of spherical scleral lenses appears to result in greater scleral asymmetry.66 However, more studies are needed to better understand the long-term effects of changes in conjunctival and scleral tissue beneath and adjacent to the landing zone or edge of soft contact lenses and scleral lenses.
Conclusions
The topography of the sclera can affect various aspects of contact lens fit, such as centration, superficial tissue compression, and optical and physiological compatibility. A detailed understanding of the anatomy and topography of the sclera as well as of possible factors influencing the scleral surface allows for an individualised selection of soft and scleral lenses. Several studies were able to show the benefits of modern measurement methods such as OCT, Scheimpflug or profilometry when determining scleral parameters. These enable a simplified lens selection, a prognosis of the lens fit and an assessment of changes in the scleral topography. Tissue compression from soft lens and scleral lens wear appears to be primarily superficial (conjunctival and episcleral) and varies with scleral topography, wear time, and lens design. Further investigations into the long-term effects of changes in the scleral topography and the regeneration time in the event of tissue compression in the area of the anterior sclera can ensure a personalised and compatible contact lens supply.
COE Multiple Choice Questionnaire
The publication "Current status of the topography of the sclera: a literature review" has been approved as a COE continuing education article by the German Quality Association for Optometric Services (GOL). The deadline to answer the questions is 1 April 2023. Only one answer per question is correct. Successful completion requires answering four of the six questions.
You can take the continuing education exam while logged in.
Users who are not yet logged in can register for ocl-online free of charge here.
396-401.