New developments in the therapy of dry and neovascular age-related macular degeneration
Purpose:
The aim of this article is to summarise currently approved and future therapeutic options for age-related macular degeneration (AMD). Further, we discuss how more detailed phenotyping of AMD using multimodal imaging will help identifying novel treatment options in the future.
Material and Methods:
We carried out a non-systematic literature review between October 1, 2022 and October 15, 2022 using the PubMed database search combinations of “age-related macular degeneration” “anti-VEGF”, “therapy”, “gene therapy” and “multimodal imaging”.
Results:
The study results demonstrate that lifestyle changes are still pivotal in the prevention of AMD. In the intermediate stages, dietary supplements may be considered and, in the future, systemic medications or laser treatments may show benefit. In the neovascular, exudative end-stage, monotherapy with anti-VEGF is now supplemented with other treatment options. Finally, regarding geographic atrophy, complement inhibition as a new type of therapy is on the verge of being approved.
Conclusion:
An exact classification of age-related macular degeneration has brought to light many different therapeutic options. Even more detailed classification of AMD according to its phenotype using multimodal imaging will hopefully better pinpoint new therapeutic options in the future.
Introduction
Age-related macular degeneration (AMD) is the most common cause of blindness in western, developed countries.1 AMD is characterised by the accumulation of extracellular deposits in the outer retina and subsequent progressive degeneration of photoreceptors and the retinal pigment epithelium (RPE) as well as adjacent tissue.2,3 Underlying these processes is a multifactorial disease involving a complex interaction of ageing, environmental risk factors and genetic susceptibility.4 The pathogenesis of AMD includes aspects such as pathological lipid deposition, chronic inflammation and complement activation, oxidative stress and impaired maintenance of the extracellular matrix. With the help of high-resolution retinal imaging, AMD can now be clinically divided into different stages.5,6 Although there have been significant breakthroughs in the treatment of neovascular AMD, there is still a lack of treatment options to prevent the progressive and irreversible degeneration of photoreceptors and the RPE that eventually leads to central vision loss.7,8 In the following article, we highlight existing and future treatment approaches for AMD for the different stages of the disease. Furthermore, this article discusses whether previous negative study results are solely based on a lack of biological efficacy, or whether the study outcomes (increase in atrophy area as well as best-corrected visual acuity) are actually suitable study endpoints for future AMD studies. Therefore, we will list possible future, alternative, structural study endpoints that may be able to identify subtle therapeutic results.
Prevention and risk factors for the occurrence of AMD
AMD is a multifactorial disease in which numerous risk factors contribute to the onset and progression of the disease.9 The early stages of AMD are characterised by the presence of drusen (fatty deposits between the RPE and Bruch‘s membrane), which can enlarge and confluence, eventually leading to the death of the surrounding photoreceptors and RPE 10 (Fig. 1). This is largely due to a disruption of the interaction between the choriocapillaris and the RPE caused by lipid deposits in Bruch‘s membrane, a thickening of the basal lamina of the RPE, basal linear deposits and the sub-RPE drusen mentioned above.11 These factors inhibit the nutrient supply to the RPE and photoreceptors. Because of this, intervention as early as possible, i.e., before the first deposits and drusen appear, should be promising. Even though many risk factors for the development of AMD cannot be influenced (e.g., genetics, age, origin, gender), certain lifestyle changes can have considerable effects.12 First and foremost, quitting smoking is worthy of mention. In large longitudinal studies (Blue Mountains Eye Study; Age-Related Eye Disease Study [AREDS]; Beaver Dam Eye study), smoking was identified as an independent risk factor with an odds ratio between 2 and 4.13,14 However, other lifestyle choices such as a Mediterranean diet, stress reduction, blood pressure regulation and abstaining from excessive alcohol consumption can also positively modify the risk of suffering AMD.15 However, we still lack therapeutic measures and interventions to prevent AMD. Considering the risk factors described above and the pathogenesis concept of the „oil spill“ of extracellular lipid-containing deposits,4 which hinder the exchange of substances between the choriocapillaris and the RPE, frequently used systemic medications such as cholesterol-lowering drugs, antidiabetics and anti-inflammatory drugs can be considered.16,17 Several epidemiological studies have already demonstrated the benefit of the aforementioned medications in the prevention of AMD.18-22 Of particular note is a recently published meta-analysis by Mauschitz et al., which, based on 14 population studies, found an association between the use of lipid-lowering substances (e.g., statins) and antidiabetics and a lower prevalence of AMD.23 However, such an association should be confirmed in intervention studies and longitudinal studies before these drugs are generally recommended as a treatment. Nevertheless, the treatment of comorbidities such as dyslipidaemia, diabetes mellitus and arterial hypertension is advisable.
In addition, several studies have shown that eye diseases such as AMD also show features of neurodegenerative diseases, and, on the other hand, CNS pathologies, such as Alzheimer‘s disease (AD) and Parkinson‘s disease, show changes at the eye level.24 This significant correlation between dementia/AD and AMD could make routine screening for the other disease useful.25 However, whether therapeutic approaches from the field of dementia/AD research, such as memantine, would also be useful for AMD requires further investigation.26,27
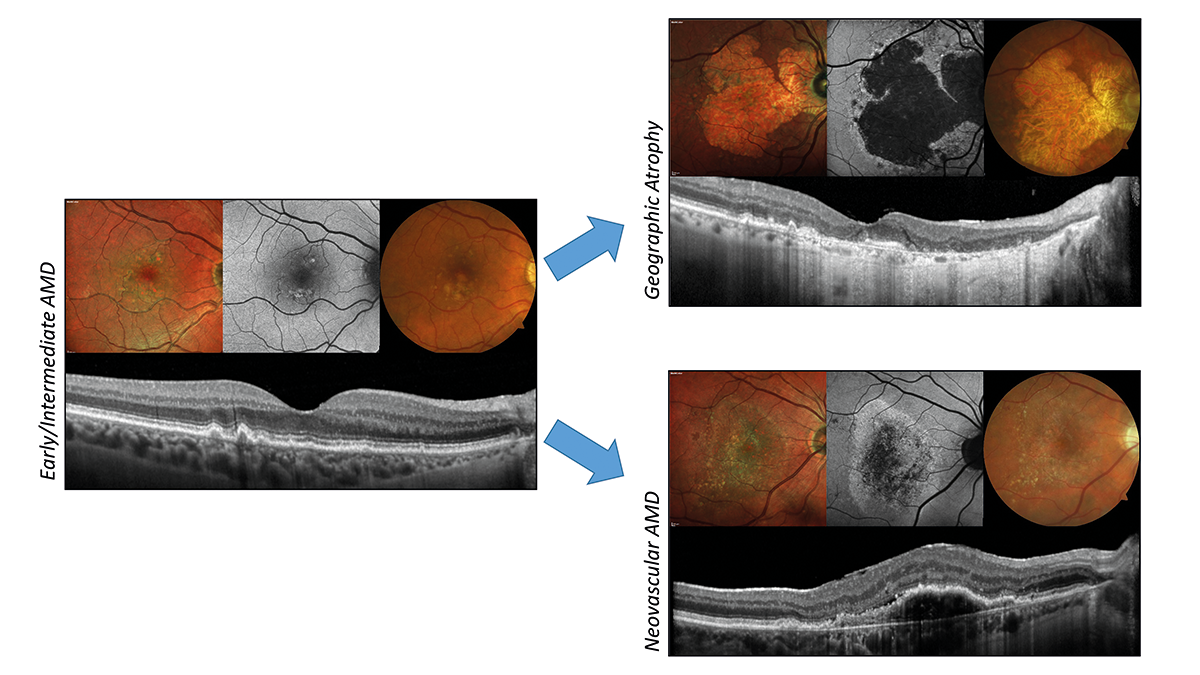
Hindering the progression of early and intermediate AMD
Avoiding that AMD progresses to the late forms (neovascular AMD and geographic atrophy [GA]) is a focus of current AMD research. The late forms are preceded by the early and intermediate disease stages, which can be detected based on drusen size and pigment changes in fundus photography.28
The disadvantage of such a rough classification is that patients can often be in the same disease stage for several years, meaning that long, interventional studies are necessary.29 Nowadays, we can identify additional biomarkers (e.g., hyperreflective foci, subretinal drusenoid deposits [reticular pseudo-drusen], nascent GA, photoreceptor band thinning, etc.) for disease progression to the late forms, especially with the use of multimodal imaging such as optical coherence tomography and fundus autofluorescence.30 So far, only two possible interventions: nutritional supplements and nanosecond laser treatment, have been shown to have a therapeutic effect.29,31 Of these two interventions, only dietary supplements with the Age-Related Eye Disease Study (AREDS) formula (antioxidants, vitamins C and E, beta-carotene and zinc) have been shown to positively influence the risk of AMD progression.31 In follow-up studies, the composition was further adjusted, as due to the increased risk of lung cancer occurrence with beta-carotene substitution, this was replaced by lutein together with zeaxanthin.31 It should be noted, however, that the effect was only demonstrated in high-risk patients with bilateral large drusen or in patients with large drusen in one eye and late AMD in the other. The nanosecond laser, on the other hand, has been shown to be effective in successful animal and preclinical studies. However, in the multicentre LEAD trial using a subthreshold nanosecond laser (SNL) to delay disease progression to late AMD, no significant difference in overall progression rate was found between subjects treated with SNL and those treated with sham therapy.29 However, in a post-hoc analysis, treatment was successful in slowing progression in patients without subretinal drusenoid deposits/reticular pseudo-drusen. Although the use of such lasers cannot yet be recommended based on the current data, the results are promising for future studies with a variation of therapy intervals, laser foci and different cohorts.
Treatment options for neovascular AMD
With the introduction of anti-vascular endothelial growth factor (anti-VEGF) therapy, visual results have significantly improved in patients with macular neovascularisation, the wet, neovascular manifestation of late-stage AMD.32 While anti-VEGF monotherapy can achieve excellent visual outcomes over 12 to 24 months, long-term outcomes are less favourable, with a loss of best corrected visual acuity (BCVA) of 15 letters or more in one third of patients.33 However, especially in recent years, the product range of modified anti-VEGF monotherapies, anti-VEGF combination therapies, anti-VEGF biosimilars and alternative forms of application has multiplied. Long-term data are still lacking for the latter in particular, but we nevertheless want to explain active substances for the various strategies in more
detail below.
Anti-VEGF monotherapy
Since the initial use of bevacizumab (Avastin; monospecific, complete antibody) in 2005, modified blockers of the VEGF cascade have been introduced. These include the humanised fragment antigen binding (Fab) fragment antibody ranibizumab (Lucentis) and the vascular endothelial growth factor receptor (VEGFR)-Fc fusion protein aflibercept (Eylea), which, unlike bevacizumab, have approval for the treatment of wet AMD.34,35 Recently, brolucizumab (Beovu), a particularly small molecule with only 26 kilodaltons (kDa, compared to 149 kDa for bevacizumab), has been approved, which promises a particularly strong and long-lasting effect.36,37
Anti-VEGF combination therapies
Faricimab (Vabysmo, 2022) is the first bispecific antibody on the market whose molecule targets both anti-angiotensin-2 and anti-VEGF-A at the same time. The addition of anti-Ang-2 is expected to stabilise the blood vessels and to have a longer effect in the eye.38 In the pivotal trials (TENAYA and
LUCERNE), faricimab reduced the median number of injections from 15 to 10 compared to the eight-weekly injection of aflibercept. In addition, for about half of the faricimab patients, intravitreal therapy was sufficient for 4 months in the first year. Longitudinal, multicentre studies must show whether faricimab will also reduce the number of injections in real-life use compared to the already established treatments. (Fig. 2)
Alternative forms of application of anti-VEGF
With the port-delivery-system (PDS; Genentech), a permanent delivery of anti-VEGF was shown to be equally effective as an alternative to repeated intravitreal injections in phase 3 studies.39 This was achieved via a permanent intraocular implant for regular drug delivery, which was surgically inserted over the pars plana and could even be refilled as needed. Due to an increased number of cases with leakage of the membrane in the PDS to the outside, the manufacturer recently put a stop to further implantations. However, the treatments already used are still being evaluated in global studies, such as the VELODROME study.40
Biosimilars
Biosimilars are biotechnological products that are comparable in terms of quality, non-clinical and clinical evaluation with an already approved reference product.41 Since patents for some of the above-mentioned treatments will soon expire or already have, there will be some movement on the anti-VEGF market. In addition, there is at least the potential to make the currently very cost-intensive therapy of neovascular AMD more affordable.
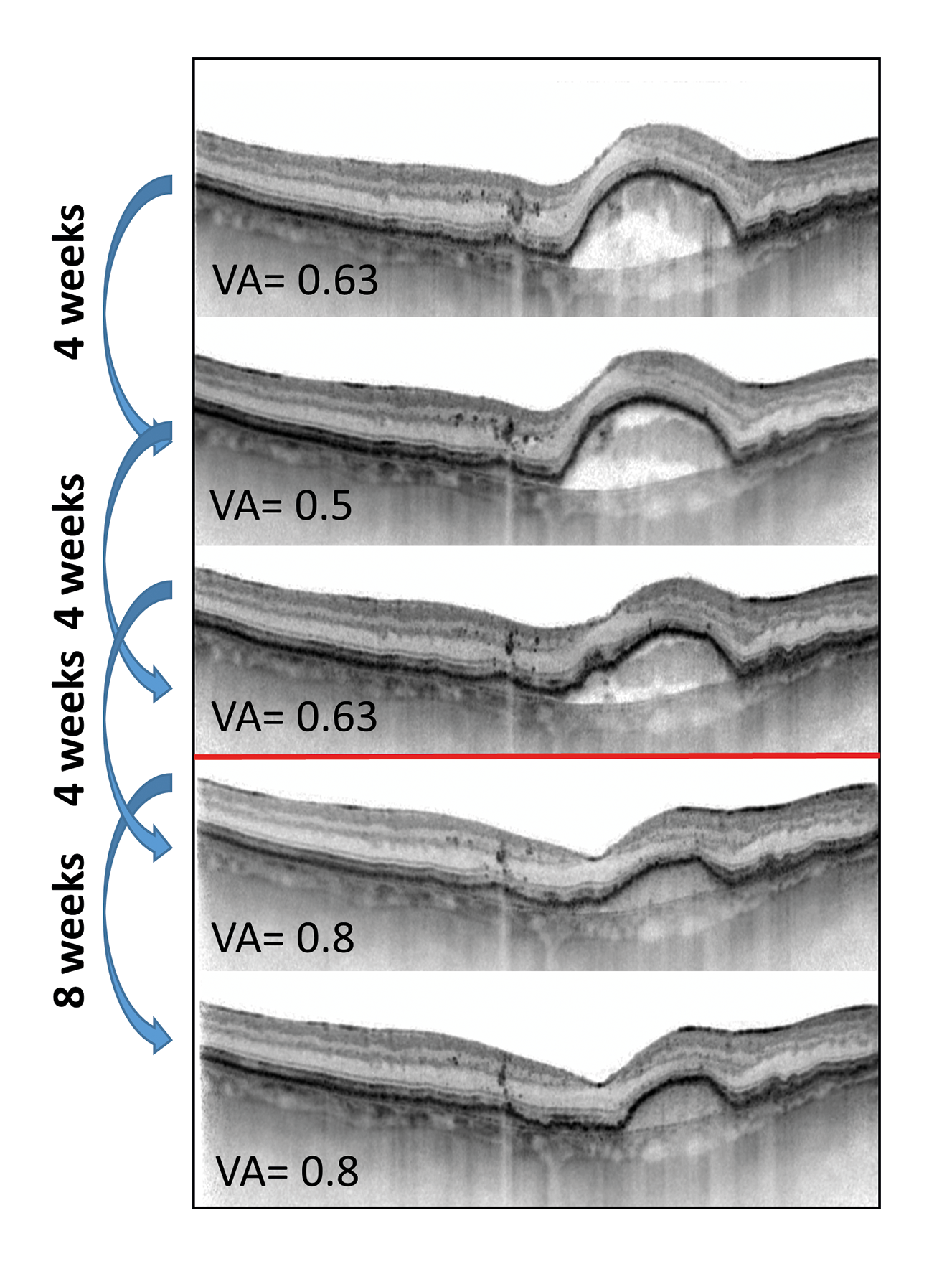
Innovative therapeutic approaches for geographic atrophy
Geographic atrophy, the dry end form of AMD, is characterised by areas of atrophy that increase in size with complete loss of the RPE and overlying photoreceptors.42 The lesions are measured using fundus autofluorescence examination and change in atrophic area is established as a clinical endpoint in interventional studies.43 However, the growth rate is slow (depending on their localisation relative to the fovea), and it is also questionable whether the marginal area of atrophy with often the most intense degenerative processes can still be saved with intervention. Thus, the threshold for potential therapeutic intervention is high.
However, additional structural and functional endpoints are increasingly being defined in order to be able to determine disease progression even more sensitively.2,44 Worthy of mention is the thinning of the photoreceptor layer, which presumably precedes RPE atrophy and whose progression forms an alternative biomarker.2
Currently, ongoing studies on GA therapy are clinically testing approaches from the fields of stem cell therapy, complement cascade inhibition, influencing mitochondrial function, influencing lipid metabolism, neuroprotection, inhibition of the visual cycle, trophic factors and cell implants.
Complement cascade inhibition and gene therapy certainly seem promising at present. The complement cascade has been discovered as a therapeutic target, as most genetic variants associated with AMD form genes for enzymes of the complement alternative pathway. After initially modest results in phase 3 studies (e.g., CHROMA and SPECTRI studies [lampalizumab inhibitor of complement factor D]), pegcetacoplan (C3 inhibitor; phase 3 studies; DERBY and OAKS) has now been shown for the first time to significantly slow GA progression (after 12 / 18 months, reduction in GA area growth by 22 % / 16 %).45–47 Approval by the US FDA is now expected soon (end of February 2023). In addition, avacincaptad (anti-C5 aptamer; phase 3 results pending; GATHER2) is another compound in the pipeline that has already demonstrated comparable results to pegcetacoplan in a phase 2 study (GATHER 1).48
Although gene therapy approaches currently focus primarily on neovascular AMD, there are also initial efforts to treat geographic atrophy. This innovative technique would be the only one of the above-mentioned therapies that offers a chance at curing the disease, for example, by replacing lost RPE and photoreceptors. In this regard, it is worth mentioning the subretinal injection of human embryonic RPE cells (phase 2, PORTRAY study), a treatment which is part of a study already in clinical trials.49 The associated phase 1 study was able to show positive results: increase in RPE layer thickness in optical coherence tomography (OCT) as well as an increase in pigmentation in the marginal area of the atrophic surface.50 Furthermore, another interesting study is the GT005 trial (FOCUS; phase 1/2), which combines complement regulation and gene therapy for the treatment of geographic atrophy.51 GT005 leads to the expression of human complement factor I, which downregulates the alternative pathway of the complement system.
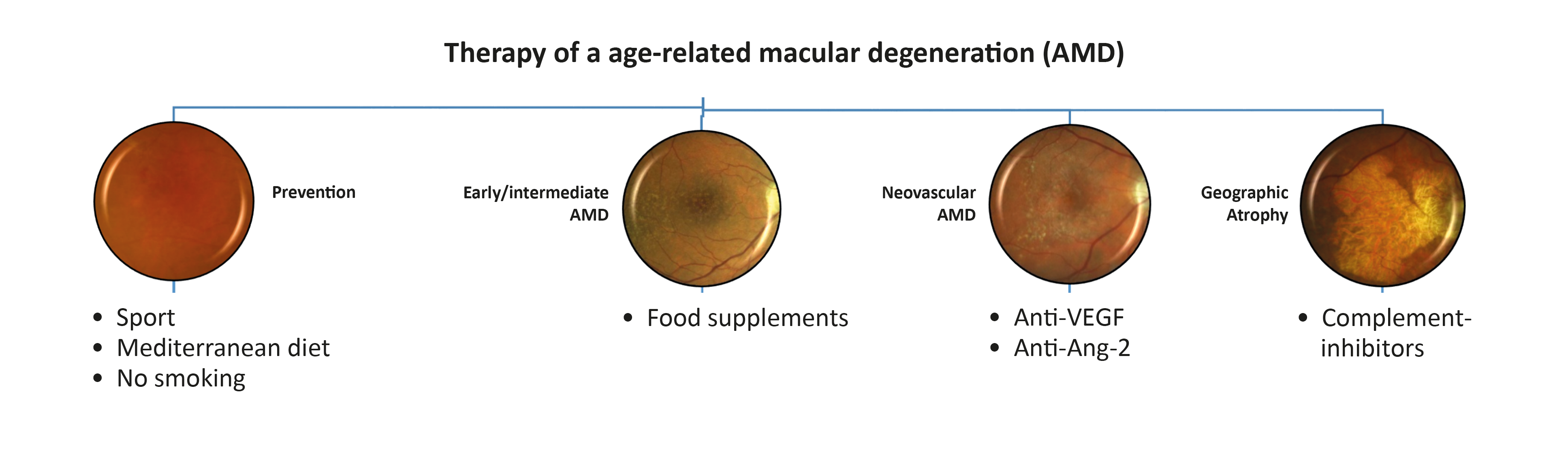
Conclusions
To date, there is only an approved therapy for wet, neovascular AMD. For the other stages (early, intermediate, late-stage geographic atrophy) there is no approved therapy so far (Fig. 3) Lifestyle changes are paramount in the prevention of AMD. In the early and intermediate forms, dietary supplements can be considered for a subgroup of patients, and in the future, systemic medications such as statins or laser therapy may also be considered. For the treatment of the neovascular, wet late stage form of the disease, anti-VEGF monotherapy can be administered with combination therapies, antibody modifications, biosimilars and alternative forms of application. Finally, in geographic atrophy, an agent has been able to show biological efficacy for the first time through the inhibition of complement cascades. Modern, high-resolution imaging with the possibility of a more precise classification and phenotyping of AMD will also help to filter out further drug therapies and their effects in the future.
Figure: Copyright by Leon von der Emde
Article for download
Download here: New developments in the therapy of dry and neovascular age-related macular degeneration
COE Multiple Choice Questionnaire
The publication "New developments in the therapy of dry and neovascular age-related macular degeneration " has been approved as a COE continuing education article by the German Quality Association for Optometric Services (GOL). The deadline to answer the questions is 01.06.2024. Only one answer per question is correct. Successful completion requires answering four of the six questions.
You can take the continuing education exam while logged in.
Users who are not yet logged in can register for ocl-online free of charge here.