Diagnosis and therapy of primary vascular dysregulations and Flammer syndrome in glaucoma patients
Purpose: Glaucoma disease leads to glaucomatous optic neuropathy (GON). However, how GON develops is still not fully understood. While increased intraocular pressure (IOP) used to be considered more or less the sole cause, we now know that there are other risk factors besides IOP. In this review we describe the importance of blood flow in GON.
Material and Methods: The literature on blood flow in glaucoma is difficult to survey. In this review we discuss publications that have contributed to the understanding of the relationship between blood flow and GON.
Results: Unstable oxygen supply leads locally to increased oxidative stress in mitochondria. This stress reduces energy production. Atherosclerosis and its risk factors lead to a more or less stable reduction of blood flow. However, in patients with primary vascular dysregulation (PVD), blood flow and thus oxygen supply are unstable. People with constitutional PVD usually have other symptoms and signs, such as low blood pressure or cold hands. The combination of PVD with the other symptoms is called Flammer syndrome (FS).
Conclusion: FS contributes to the development of GON since it interferes with the autoregulation of ocular blood flow. Thus, blood pressure and IOP fluctuations lead to an unstable blood flow and to an unstable oxygen supply. Consequently, oxidative stress increases, which contributes decisively to GON development.
Introduction
Blood is an important transport vehicle and a perfect blood circulation is a prerequisite for the functioning of all organs. Since there are no oxygen reserves in the tissues, this supply must be continuous. To ensure this, we have a heart that pumps blood constantly and an extensive network of large and small blood vessels with a total estimated length of 150.000 km. There are many causes and forms of circulatory disorders which influence the occurrence and progression of diseases. This review provides an introductory overview of the role of blood flow in glaucoma. For further details and to access the publications cited in this review, please refer to the Ocular Blood Flow Working Group of the Swiss Glaucoma Research Group: www.glaucomaresearch.ch
Causes of Glaucomatous Optic Neuropathy (GON)
Glaucoma was previously understood to be a disease related to intraocular pressure (IOP). An elevated IOP mechanically damages the optic nerve head, leading to excavation and visual field loss. Accordingly, glaucomas were classified according to the respective causes of IOP increas.This understanding of the disease has now changed, because GON can occur without elevated IOP and, in many cases, an elevated IOP does not lead to GON.1 Thus, it is clear that other risk factors (RF) and mechanisms contribute to the development of GON. However, experts struggle to find and understand these factors. Some papers discuss mechanical factors, such as anatomic weakness, e.g. in myopia or too low intracranial pressure. The A potential causal role of autoimmunity, which is often present, is also being discussed. Vascular causes were suspected very early on, but at the same time always questioned. Many studies investigated the relationship between glaucoma and atherosclerosis or its RF, but the results were rather disappointing and partly contradictory. While some studies showed that, for example, arterial hypertension or diabetes mellitus increase the risk of GON, others showed that they actually decrease the risk of GON.2,3,4 Today, we know that all RFs of atherosclerosis, such as smoking, obesity, dyslipidemia, diabetes, or arterial hypertension, are weak but significant RF for an increase in IOP and thus promote the development of GON. Beyond that, however, their direct influence on GON is surprisingly small. A breakthrough came with the realisation that it is not so much atherosclerosis and its RF, but rather a malfunction of the regulation of blood flow that directly contributes to the occurrence or progression of GON. The lower the IOP at which GON occurs or progresses, the more frequently vascular dysregulation is found. Correspondingly, its importance is particularly large in normal tension glaucoma (NTG). Atherosclerosis leads to a more or less steady decrease of blood flow in the eye whereas, in vascular dysregulation, blood flow fluctuations increase significantly. Neural tissue can adapt much better to a constant oxygen reduction than to oxygen fluctuations.
The regulation of ocular blood flow
Until the 1970s, the regulation of blood flow seemed to be simple: the blood vessels were considered to be relatively stable structures. Regulation was controlled mainly by the autonomic nervous system and took place mostly in the small precapillary arteries (arterioles). During physical activity or emotional stress, the sympathetic tone increased, which increased the heart rate and, at the same time, tightened the arterioles, which led to an increase in blood pressure. In the 1980s came the surprising discovery that it is mainly the innermost layer of the blood vessels, the endothelial cells, that regulate all the blood vessels, from the arteries to the capillaries and the veins. Because the influence of endothelial cells, unlike the influence of the autonomic nervous system, is very local, there may also be local differences in the transient dilation or constriction of blood vessels. During those years we studied isolated ocular blood vessels with myographs and perfused isolated eyes with the Langendorf model. We could show that the vessels of the eyes are also regulated by these endothelial cells in that these cells permanently release messenger substances, such as NO and endothelin, and thus very locally regulate the vessel width and the flow resistance.5 The magnitude of the blood flow results from the dynamic relationship between perfusion pressure and local resistance. Whereas in the choroid the autonomic nervous system is a dominant regulator, the endothelial cells are the dominant ones in the non-innervated vessels of the retina and optic nerve head.
Dysregulation of ocular blood flow
Fankhauser and Bebie developed automated perimetry in the 1970s. Based on this, we developed the visual field indices, the glaucoma programmes G1/G2 and the Bebie curve in the 1980s. Equipped with these methods, we made the observation that the differential light sensitivity fluctuates over time and that the long-term fluctuation is significantly greater in glaucoma patients than in healthy people. We also observed that visual field loss can be provoked in certain patients (e.g., by the cold) and that the glaucomatous visual field defects were partially reversible under certain treatments. This reversibility depended less on the effect of these drugs on IOP than on their effect on blood flow. In parallel to the visual field improvements and deteriorations, we observed improvements and deteriorations of blood flow in the nail fold capillaries in the same patients. Thus, it became clear that certain glaucoma patients have a faulty vascular regulation both in blood vessels in the fingers as well as in the eyes.5 Since the angiologists explained such phenomena with as vasospasms or with a vasospastic syndrome, we adopted this terminology in our preliminary publications6, but then noticed that these patients did not only have spasms, but rather a complex regulation disorder. To better describe this, we introduced the term vascular dysregulation into the literature. Vascular dysregulations are either a result of a disease or the consequence of a predisposition, which we named secondary vascular dysregulation (SVD) and primary vascular dysregulation (PVD), respectively.7 While SVD can lead to non-glaucomatous optic atrophy, PVD is an RF for GON.
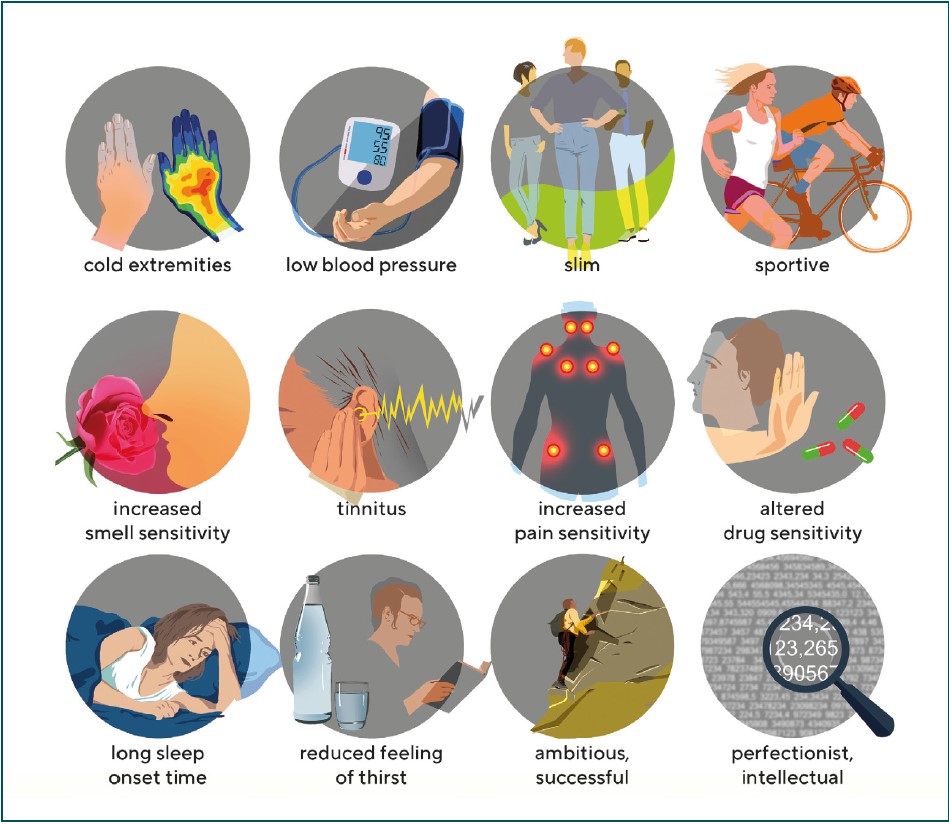
Flammer syndrom (FS)
Having identified a link between PVD and GON, we found a number of other commonly associated signs and symptoms in affected patients. Figure 1 illustrates some of these symptoms and Tab. 1 lists the most important signs. An international and interdisciplinary group of specialists then named Flammer Syndrome (FS) the association of PVD with these symptoms.8 FS plays a role not only in GON, but also in other diseases9, see: www.flammer-syndrome.ch The reason why the blood vessels in subjects with PVD/FS behave differently than in healthy people is mainly due to the altered behaviour of the endothelial cells. However, we must distinguish this endotheliopathy in FS from the endotheliopathy that we see in the context of arteriosclerosis and its RF.10
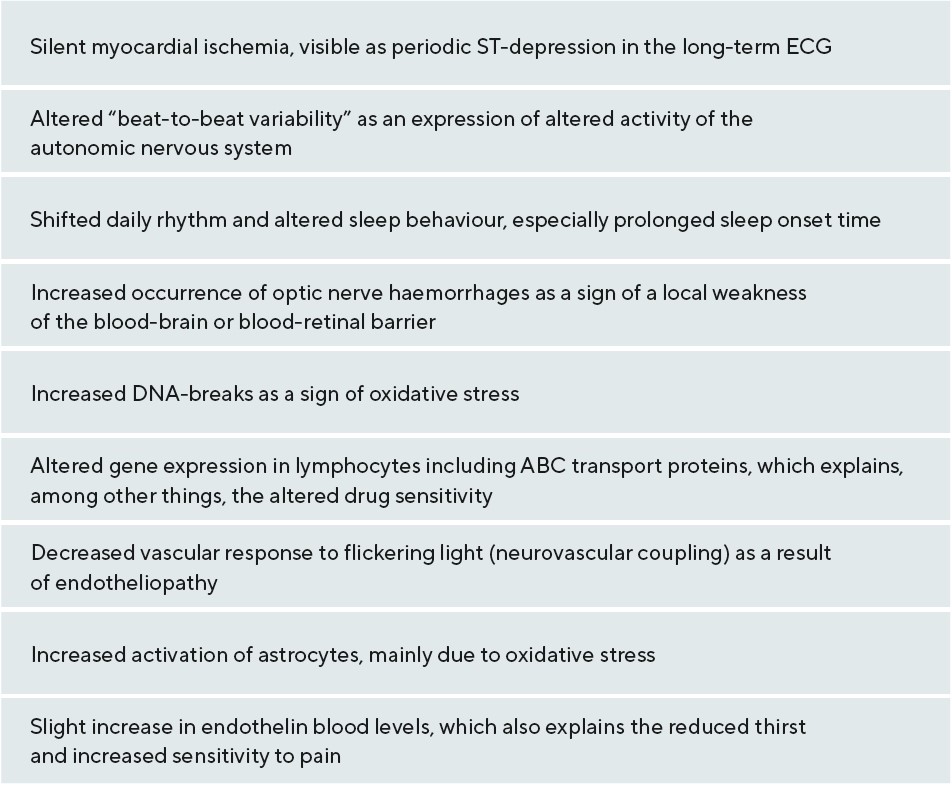
Reduction and fluctuations in oxygen supply do not have the same consequences
When the oxygen concentration is low, e.g., in the case of an optic disc infarction, the neuronal cells die. This leads to a pale, non-excavated optic disc. The reason lies in the behaviour of the glia, especially the astrocytes. These not only survive hypoxia, but they also even proliferate and form a glial scar. However, these astrocytes react quite differently to oxidative stress. They are also first activated initially, but and then die over a longer period of time. If both the nerve cells with their axons and the astrocytes die, the optic nerve head excavates11, a phenomenon we call GON, as illustrated in Figure 2. Oxidative stress can occur very locally, e.g., as a result of a local unstable oxygen supply. In the respiratory chain of the mitochondria, the electrons always appear as pairs with opposite spin. These pairs migrate step by step towards oxygen according to the energy gradient. If the oxygen concentration drops, the electrons accumulate and at the moment when the oxygen supply rises again, some of them jump individually on to oxygen molecules and form a superoxide, i.e. a free oxygen radical.12 There are various reasons for unstable oxygen supply, e.g., sleep apnoea or a severe drop in blood pressure. A common cause is FS. People with FS usually have low blood pressure, increased retinal venous pressure (RVP) and an inability to compensate for this by regulating the blood vessels.5,13
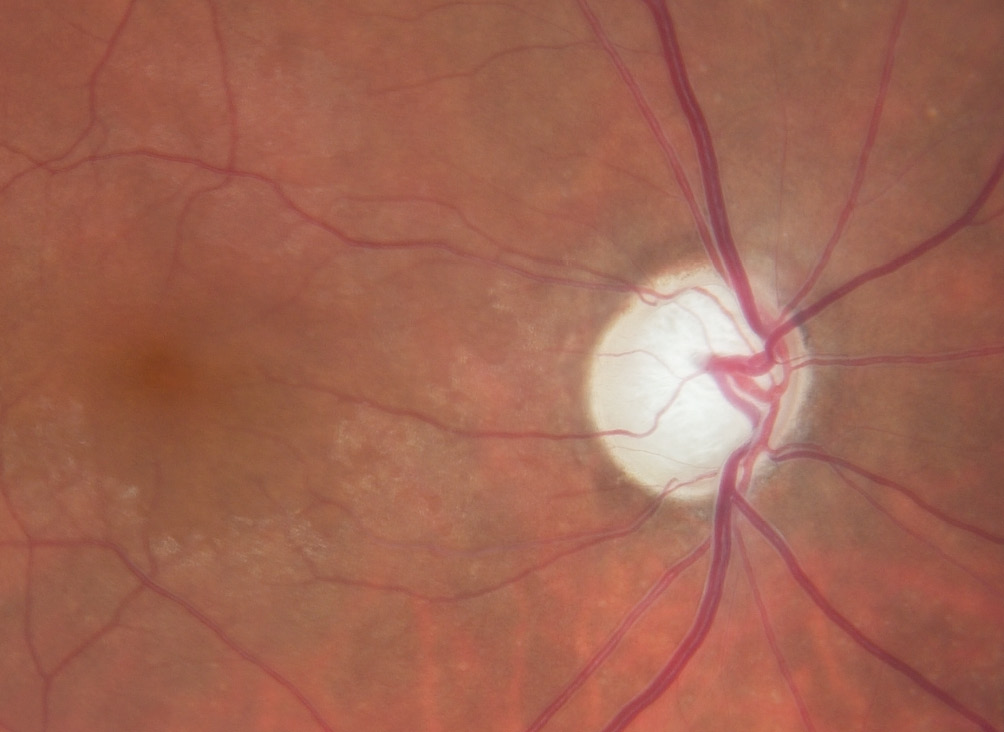
Perfusion pressure (PP) is also dependent on retinal venous pressure (RVP)
Based on studies in healthy animals, it was previously assumed that RVP was the same as IOP. Accordingly, PP was calculated from the difference between retinal arterial pressure (RAP) and IOP. RAP was estimated based on blood pressure measured on the arm. We know today that such an estimate of RAP is inaccurate and, more importantly, that RVP is often higher, sometimes markedly so, than IOP, especially in people with certain ocular or systemic diseases.14 This is also true for many glaucoma patients, especially those with NTG and FS.15 Accordingly, in these patients perfusion pressure is lower than previously thought.14 Autoregulation in the retina and optic nerve head ensures that perfusion is independent from PP to a certain extent. If this autoregulation is disturbed, every change in PP also affects the blood flow. If the intraocular pressure or the blood pressure fluctuates in these patients, then the blood flow also fluctuates and, with it, the local oxygen concentration. Because people with FS have a disturbed autoregulation, their oxygen supply in the retina and the optic nerve head is unstable. The result is locally increased oxidative stress.
Diagnostic consequences
We must look for other causes if GON occurs or progresses despite normal IOP or normalized IOP. A systemic history, especially considering possible symptoms of FS, already leads us in the direction of further investigations. A 24-hour blood pressure measurement can detect drops in blood pressure. An increased retinal venous pressure is a clear indication of a circulatory disorder and can be measured easily and quickly14, e.g., with an ophthalmodynamometer16. In certain cases, further vascular investigations are possible or even necessary.5
Therapeutic consequences
Many mechanisms are involved in the development of GON. These rarely lead to damage on their own, but usually do when in mutual interaction.11 The weaker the vascular regulatory capacity, the smaller the tolerance for intraocular pressure peaks or blood pressure drops. This means that we can intervene therapeutically in various mechanisms. On the one hand, the question arises as to which mechanism is particularly prominent in a specific patient, and, on the other hand, which therapy options are available or which therapies can the patient tolerate. The more advanced the GON and the longer the life expectancy of the patient, the more likely it is to use different therapies simultaneously. In the case of elevated IOP without GON (ocular hypertension), lowering the IOP is the only treatment. Above which IOP such a treatment makes sense must be decided individually. Today, there is a tendency to over-treat rather than under-treat. If GON has developed under elevated IOP, then lowering the pressure always makes sense. If the damage is already far advanced or if it continues to progress despite normalised IOP, then additional therapies are necessary. If GON has occurred at normal pressure (NTG), a further lowering of the IOP is usually still recommended, but the focus must lie on other treatments. Depending on the individual findings, we should pursue the following goals: improving the regulation of ocular blood flow, lowering an increased RVP, preventing blood pressure drops and reducing oxidative stress. A combination of magnesium with a very low-dose calcium channel blocker has proven effective in improving vascular regulation; increased salt intake in reducing blood pressure drops; and ginkgo biloba, among others, in reducing oxidative stress.17 The reduction of increased RVP is particularly important. We observed that retinal vein occlusion in some patients was not primarily caused by thrombosis but by local constriction of the veins18 and that endothelin-induced local constriction are the main cause of RVP increase.19 This fits with the observation that endothelin blockers decrease RVP.20 Magnesium, low-dose calcium antagonists and antioxidants also have a beneficial effect on RVP.21 The effect of a special vitamin preparation containing L-methylfolate (Ocufolin® forte) is interesting. It lowers RVP22, reduces oxidative stress, improves retinal blood flow23, lowers homocysteine levels24 and also slightly lowers IOP. How to specifically proceed and when to use which therapy was recently described in detail in another a review.17
Conflict of interest
The authors have no conflict of interest with regard to the methods and devices mentioned in the article.
Abbreviations:
GON: Glaucomatous Optic Neuropathy
IOP: Intraocular Pressure
PVD: Primary Vascular Dysregulation
SVD: Secondary Vascular Dysregulation
FS: Flammer Syndrome
RF: Risk Factors
NTG: Normal Tension Glaucoma
PP: Perfusion Pressure
RVP: Retinal Venous Pressure
RAP: Retinal Arterial Pressure